The Spitzer Space Telescope has spent the best part of two decades scanning the Universe in infrared, revealing the secrets of the cosmos with its unique view of star-forming regions and ancient galaxies.
But the story of the Spitzer Space Telescope's legacy goes back much further.
Shortly after the Second World War, a young physicist from Yale University published a report outlining the advantages of putting a telescope into space.
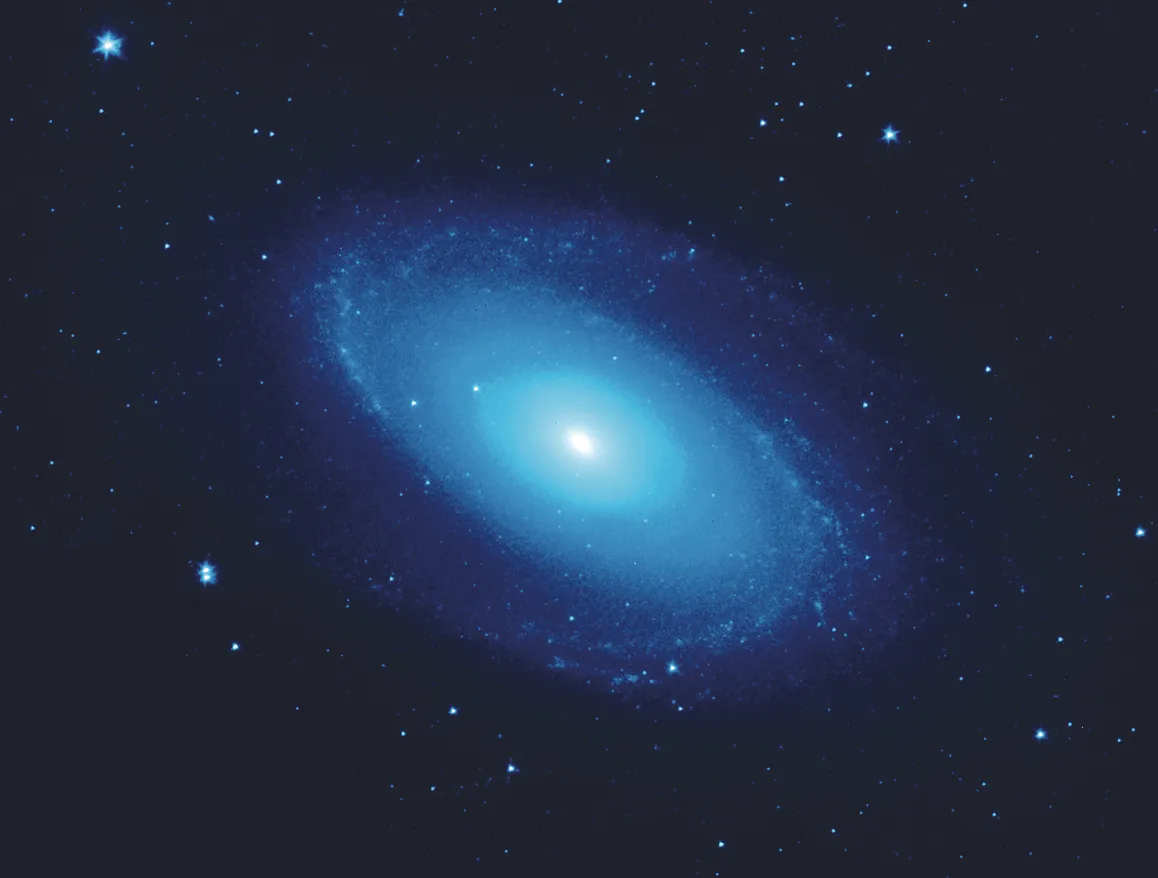
High above the blurring effect of Earth’s atmosphere, he wrote, it could discern the faintest stars and the furthest galaxies with crystalline clarity.
His 1946 paper illustrated a growing appreciation for how space travel – still a decade away from becoming reality – could revolutionise astronomy.His name was Lyman Strong Spitzer.
Read more about the Spitzer Space Telescope:
The infrared telescope bearing his name has transformed our understanding of how stars are born and die, how planets evolve and thrive far beyond the Solar System and what lies at the very heart of our Milky Way Galaxy.

Spitzer’s name was not simply plucked from a hat, when NASA decided its sparkling new observatory needed a catchier eponym than the unwieldy ‘Space Infrared Telescope Facility’ (SIRTF) it had borne since its inception two decades earlier.
As well as being one of the foremost astronomers of his generation, Spitzer (who died in 1997, aged 82) contributed hugely to studying the interstellar medium, the ubiquitous gas and dust from which new stars form, and his enthusiasm for an Earth-circling astronomical eyepiece eventually won support for the Hubble Space Telescope.

Hubble is one of four ‘great observatories’ to scrutinise the heavens in unrivalled detail; another, the Compton Gamma Ray Observatory, examined high-energy photons, while a third, the Chandra X-ray Observatory, did likewise for X-rays.
The final sibling, SIRTF, was originally earmarked to fly on the Space Shuttle. But the reusable spacecraft’s relatively ‘dirty’ environment – constantly emitting vapours, particles and heat – made it unsuitable for sensitive infrared optics whose raison d’etre was detecting thermal radiation.
Plans correspondingly changed and SIRTF evolved into an orbiting satellite with high priority in the field of space-based astronomy.
But it was also horrendously expensive. Various scenarios to launch it on Titan, Atlas or Delta rockets threatened to diminish its scientific integrity and its cost hovered around two billion dollars, four times higher than NASA wanted to spend in the fiscally lean years of the 1990s.
Then came a breakthrough. By putting SIRTF into an ‘Earth-trailing’ orbit, it would drift away from us at 14.9 million km every year, ridding itself of the negative thermal effect of our planet on its sensors and winning NASA and prime contractor Ball Aerospace plaudits for saving hundreds of millions of dollars.
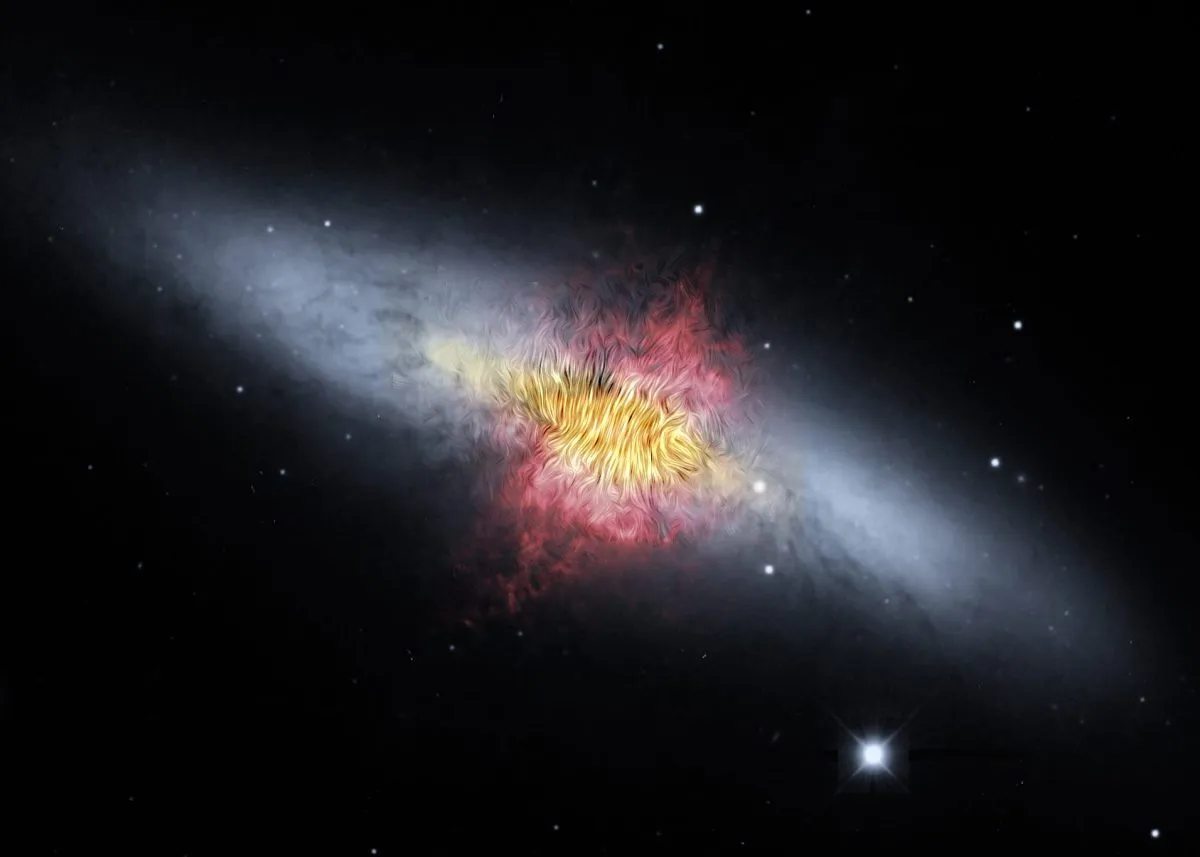
Seeing infrared
Infrared astronomy has been practised for two centuries, although it was sparsely explored until recently.
Telescopes aboard high-altitude balloons, aircraft and sounding rockets could rarely get high enough to make effective observations.
But in 1983, the Infrared Astronomical Satellite (IRAS) found 350,000 new objects and peered for the first time into the blazing heart of our Milky Way.
The demand for infrared missions quickly gained traction. In the mid-1990s, the Infrared Space Observatory (ISO) detected water vapour in star-forming regions and embryonic planets growing around dying stellar remnants.

More recently, the Herschel Space Telescope shed new light on how stars are born and revealed that much of Earth’s water probably originated from comets; indeed, it demonstrated that Comet Shoemaker–Levy 9 had delivered water into Jupiter’s atmosphere.
The Wide-field Infrared Survey Explorer (WISE) and AKARI missions also found thousands of new comets, the first near-Earth trojan asteroid and the infrared ‘fingerprints’ of a supernova, 200,000 lightyears away, deep inside the Small Magellanic Cloud.
5 of Spitzer's most jaw-dropping images
Keeping cool
To attain thermal sensitivity in the infrared, telescopes need to cool their optics, lest their measurements become contaminated by radiated heat from Earth or their own electronics.
Large cryostats of liquid helium can accomplish this, but when the cryogen runs out the telescope rapidly warms up, its sensitivity to thermal infrared radiation declines and its effectiveness vanishes.
Spitzer’s Earth-trailing orbit eliminated the problem of our planet’s heat and laid the groundwork for another cost-saving revolution: the ‘warm-launch’ mission architecture.
Rather than encasing the whole spacecraft into a cryostat – and increasing its size, weight and cost to boot – only Spitzer’s infrared sensors needed to be chilled before launch.
The rest could be built and tested at ambient temperatures. This made it simpler, cheaper and less complex and allowed it to fit a smaller Delta II rocket and carry a smaller cryostat.
And when it reached deep space, with no heat-emitting Earth to worry about, Mother Nature (and a built-in sunshade) could passively cool it to –271°C.
The result was that while the Earth-circling IRAS needed 114 gallons of liquid helium to keep cool and lasted 10 months, Spitzer carried 95 gallons but could endure for many years.
The clever choice of orbit and warm-launch architecture brought project costs tumbling to $800 million, while parallel technological advances saw infrared sensors evolve from a few dozen pixels per detector on IRAS to 65,000 pixels per detector on Spitzer.
Also, the spacecraft was built from lightweight beryllium, which cools rapidly, and a ‘store-and-dump’ method of data transfer meant it spent less time relaying its findings back home and more time doing scientific research.
Launched from Cape Canaveral on 25 August 2003, the 865kg telescope sailed through initial checks and its three instruments – the Infrared Spectrograph (IRS) and Multiband Imaging Photometer for Spitzer (MIPS), both built by Ball Aerospace, together with NASA’s four-channel Infrared Array Camera (IRAC) – came alive.

For the next 16 years, Spitzer improved our comprehension of how stars and planets form, including distant ‘extrasolar’ worlds.
It found newborn stars in the Tarantula and Trifid Nebulae, revealed infant stars growing within supernova remnants and showed that even long-dead stars such as Cassiopeia A still punch out periodic bursts of energy.
It discovered supermassive black holes hidden in luminous clouds of gas and dust, studied the merger of the Antennae galaxies and under its infrared gaze hundreds of thousands of ancient stars and masses of younger ones blazed furiously at the Milky Way’s core.
It traced some of the most ancient events since the dawn of creation, with GN-z11 in the constellation Ursa Major now recognised as the oldest and most distant known galaxy in the observable Universe, dating back some 13.4 billion years, not long after the Big Bang.
Spitzer found evidence of organics in the form of polycyclic aromatic hydrocarbons billions of years older than our Solar System, as well as partial ingredients for DNA and protein around the star IRS 46.
It showed that planets evolve around the most improbable of hosts, including brown dwarfs and hypergiant stars where conditions were previously considered too inhospitable.
And Spitzer underscored precisely how inhospitable these extrasolar worlds are.
It directly measured light emitted by the ‘hot Jupiters’ HD 209458b and TrES-1, revealed surface temperatures of 840°C and roaring 9,650km/h winds on HD 189733b.
It showed the tidally-locked planet Upsilon Andromedae b has a thermal differential between its two hemispheres of 1,400°C – a hellish place that one scientist likened to jumping into a volcano.
The spacecraft determined that protoplanetary discs can slow their parent star’s rotation, found the Sun’s close neighbour Epsilon (ε) Eridani to have not one, but two asteroid belts, and raised the likelihood that there are many worlds in the Universe with two suns, just like Luke Skywalker’s Star Wars home, Tatooine.

End of the line
In May 2009, Spitzer’s coolant ran out and temperatures rose to –242°C: still chilly, but not chilly enough for IRS and MIPS to function.
Still, the two short-wavelength channels of IRAC remained active and NASA recalibrated the spacecraft for an extended ‘warm’ mission.
For another decade, the flood of discoveries continued: finding Saturn’s tenuous Phoebe ring, scrutinising the rocky exoplanet HD 219134b, discovering the trans-Neptunian object Sedna and observing Earth-sized worlds in the habitable ‘Goldilocks’ zone around the cool red dwarf TRAPPIST-1.
But as its orbit carried it further from Earth – more than 257 million km away as 2019 ended – the ageing Spitzer had to pitch at higher angles to transmit data with a corresponding reduction in the amount of incident sunlight on its solar panels.
On 30 January 2020, when its mission ends, the Spitzer team can reflect with pride on a spacecraft that tripled its predicted lifetime and infinitely exceeded all expectations.
And like Lyman Strong Spitzer himself, whose extraordinary life ended after an ordinary day at work, so his mechanised namesake will take its place in history with grace and dignity.
What next for infrared astronomy?
When Spitzer falls silent in January 2020, another year will pass before the infrared baton of discovery passes to NASA’s James Webb Space Telescope.
It was hoped that Webb’s early days in space would happily coincide with the final days of Spitzer, but technical troubles conspired against a neat overlap between them.
Webb’s launch is currently scheduled for March 2021, and when it does so it will bring four powerful infrared instruments to bear on some of the thorniest problems in astronomy.
It will observe the earliest stars and galaxies, study how they and their planetary retinues evolved and, in the case of our Solar System, how the ingredients for life came to arrive here.

Europe’s Euclid infrared observatory will follow in June 2022 and seeks to look back over 13.5 billion years to investigate how the Universe expanded and how the first galaxies formed.
It will observe billions of astronomical sources with a precision 50 times finer than is presently achievable with ground-based instruments.
And in the mid-2020s NASA’s Wide Field Infrared Survey Telescope (WFIRST) – whose fate still rests on a knife-edge following efforts by the Trump administration to cancel it – will work in tandem with Euclid to tackle basic questions about dark energy in the Universe, as well as taking a census of exoplanets and acquiring the first direct images and spectra of them.
This article originally appeared in the January 2020 issue of BBC Sky at Night Magazine. Ben Evans is a space journalist and writer.
An interview with Spitzer's lead scientist
Dr Michael Werner has been lead scientist on the Spitzer Space Telescope mission since 1984 and his book, More Things In The Heavens, reveals how important infrared astronomy has been to increasing our understanding of the cosmos.
BBC Sky at Night Magazine spoke to Dr Werner to discuss the values of infrared astronomy and the legacy of the Spitzer Space Telescope.
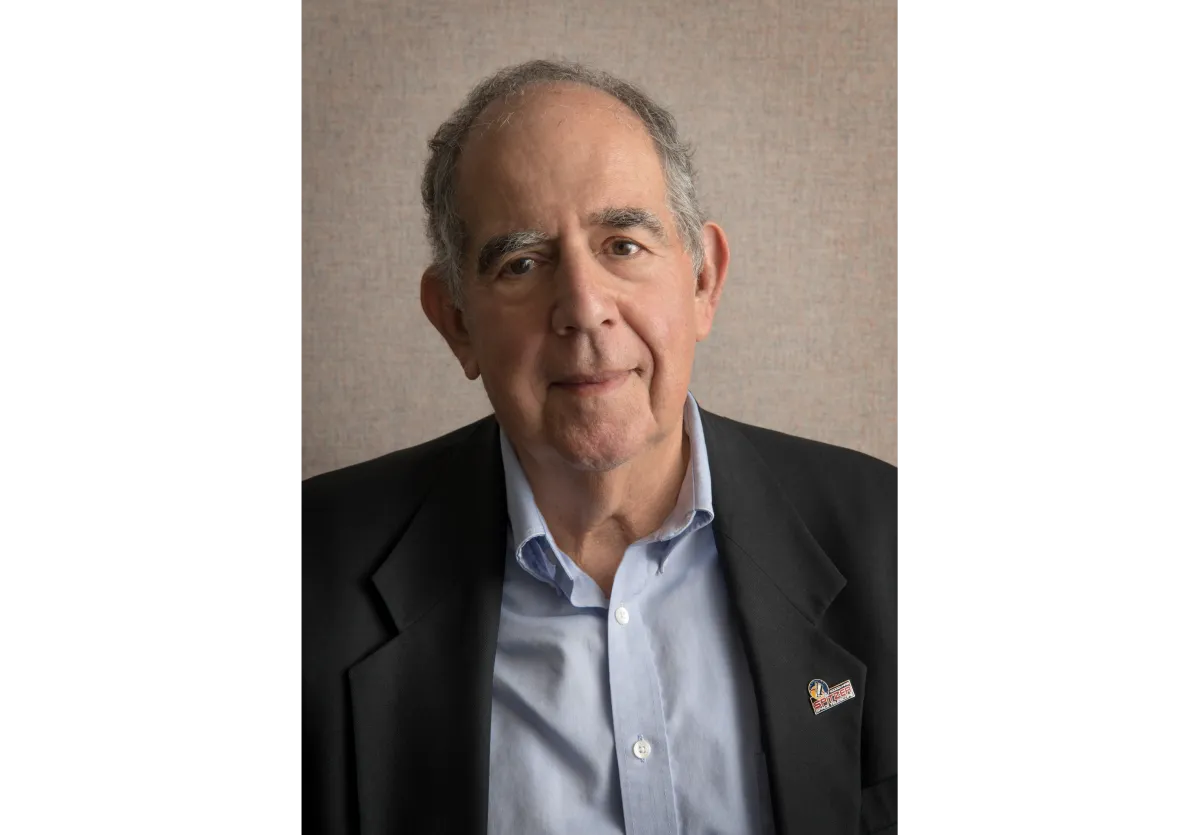
When did astronomers first realise the benefits of infrared astronomy?
The technical difficulties of detecting infrared radiation caused infrared astronomy to lag behind studies of the Universe at visible wavelengths.
Infrared work began in earnest in the 1960s using instruments on large ground-based telescopes, such as the famed 200-inch telescope at Mount Palomar.
Others sent telescopes into the upper atmosphere on balloons or high-flying aircraft.
Although there had been very substantial previous work, I think it was the IRAS all sky survey in 1983 that really showed to the entire community the power of the infrared for astronomical investigation.
IRAS achieved over-the-entire-sky sensitivity levels in the infrared that previously had been accomplished only on a few very limited areas, cataloging hundreds of thousands of sources in the infrared.
These included forming stars, galaxies, post-main sequence objects and on and on.
IRAS’s discovery of dust discs around main sequence stars, now known to be a signal of exoplanetary systems around these stars, presaged the tremendous explosion of the study of exoplanets that has becomes Spitzer’s forté.
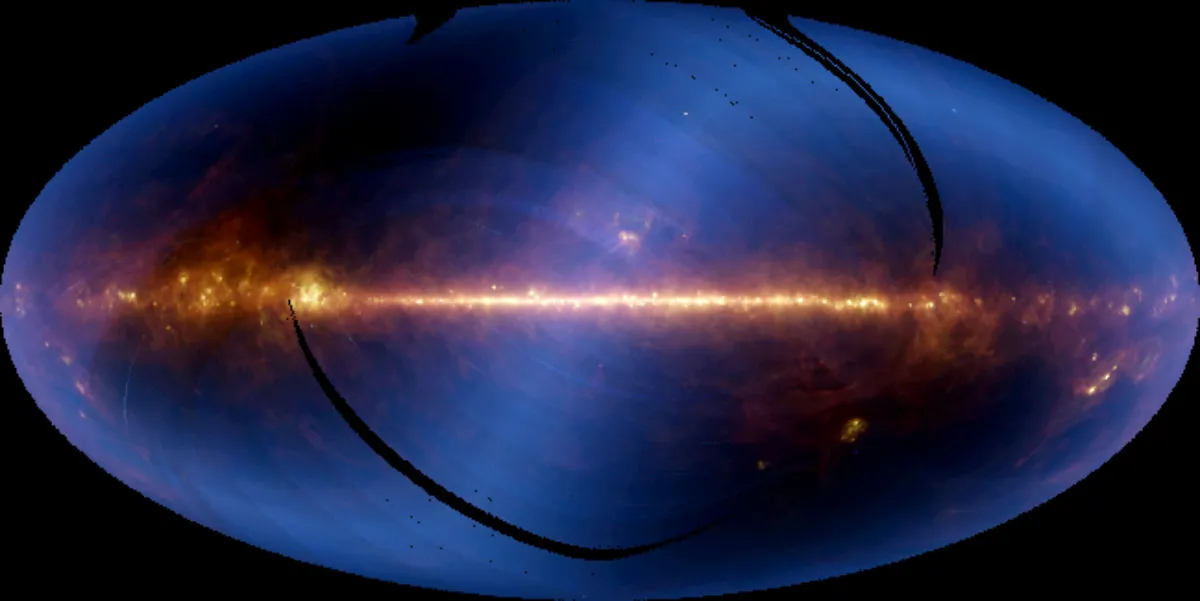
What does infrared reveal about the Universe that optical light does not?
We used to summarise infrared astronomy as “the old, the cold and the dirty”.
The "old" because the cosmic expansion redshifts light from distant objects into the infrared. This is some of the oldest light in the Universe because it has been travelling towards us for billions and billions of years.
The "cold" because in the infrared we can see objects that are too cool to radiate much visible light.
In practice this means cooler than ~2000K or so and includes brown dwarf stars, interstellar or circumstellar matter, planets and exoplanets.
The "dirty" because much of the infrared light that we see is radiated by particles of interstellar and circumstellar dust, and also because dust clouds that are opaque at visible wavelengths may be quite transparent in the infrared.
How did Spitzer come to be? Was it a difficult project to get off the ground?
Spitzer began life in the early 1970s as a proposed attached payload to operate from the bay of the Space Shuttle and to return to Earth after a few weeks for replenishment and installation of new instruments.
Its evolution from this concept into the elegant, compact system that has operated from solar orbit for over 15 years was indeed tortuous and often difficult.
What kept us going and made it all worthwhile was the huge gain in capability we envisioned [and realised] from putting infrared detector arrays into space on a cold telescope.
A major milestone along the way was the designation of Spitzer as the highest priority new space mission by the 1990 decadal review of priorities for US astronomy and astrophysics.
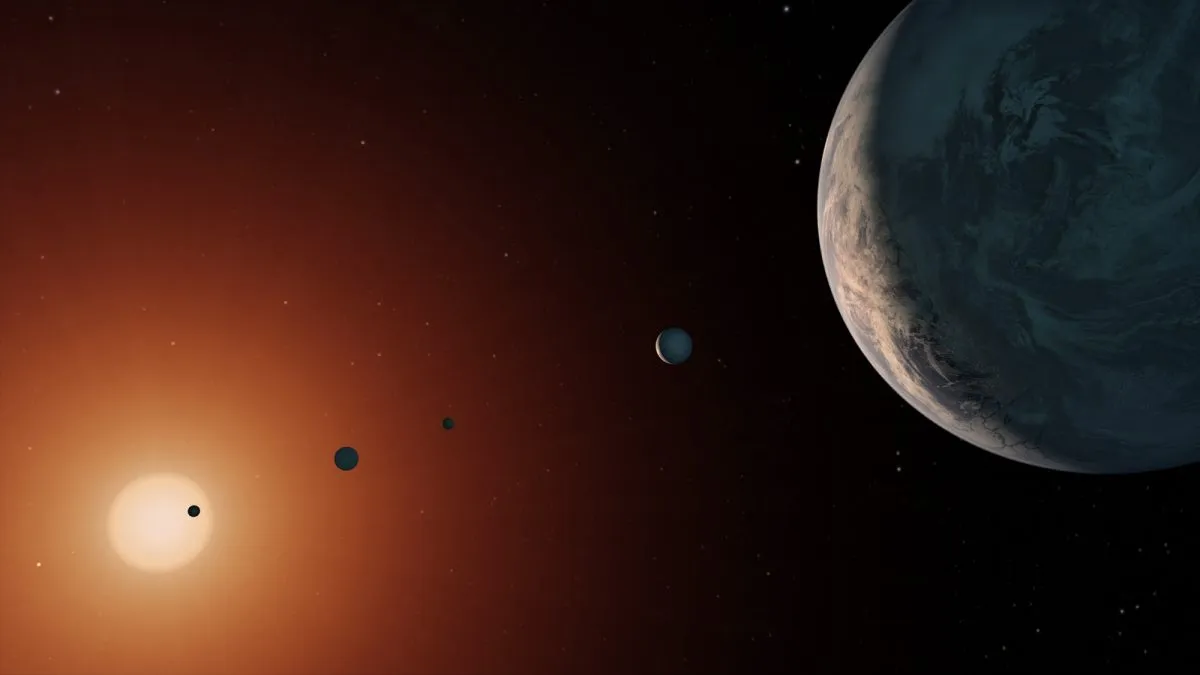
What do you think have been Spitzer’s highlights?
First there was the identification of seven Earth-sized exoplanets - planets around stars beyond our Solar System - orbiting the same faint red star now known as TRAPPIST-1.
Three of these exoplanets are in the 'habitable zone', the region around this star where water would be liquid on the surface of a rocky planet, which is thought to be a prerequisite for the formation of life as we know it on Earth.
Second has been the identification and characterisation (often jointly with the Hubble Space Telescope) of very distant galaxies, which we see when the evolving Universe was only 3 per cent of its current age and about 8 per cent of its present size.
I think that most Spitzer scientists would agree on the above; beyond which personal choice is at play.
I personally like the discovery of a giant new ring around Saturn; the identification of the C60 molecule or 'buckyball' in space; the measurements of winds and the studies of energy transport processes in the atmospheres of exoplanets; the determination that planet formation is well under way within a few million years of the initial collapse of a forming star; and showing the many similarities – in composition, architecture, and dynamic processes - between our Solar System and exoplanetary systems.
This allows insights from our own Solar System to be used in the study of exoplanetary systems, and vice versa.
Spitzer has enabled the study of the cosmic history of star formation from the earliest observable epochs to the current day.
Dr. Michael Werner is Spitzer Space Telescope Project Scientist and Chief Scientist for Astronomy and Physics at the Jet Propulsion Laboratory, California Institute of Technology.