The realisation that light could harbour information about celestial objects came thanks to the work of the German glass-maker Joseph von Fraunhofer. In 1814 he dispersed the Sun’s light into a rainbow of colours – known as a spectrum – discovering numerous dark absorption lines that were later found to betray the composition of our star.
With the subsequent development of spectroscopes to view – and spectrographs to record – celestial spectra and the lines they contained, astronomers had a means to examine the make-up of stars.
Ever since those early days, spectroscopy and the spectrograph have been an integral part of astronomical research, giving astronomers insights into not just the composition of faraway bodies but also their temperature, distance and even movements.
Key to understanding why spectroscopy is such a formidable tool for astronomers is knowing what creates the features of a spectrum.
Read more about spectroscopy:
- Getting started in spectroscopy
- NASA's CASE instrument added to ESA's ARIEL mission
- New technique could discover life on Mars
You can think of the lines in astronomical spectra as celestial fingerprints, in that they can help scientists work out what atoms and molecules created them.
These ‘fingerprints’ come in two forms, absorption lines and emission lines. Let’s look at absorption lines first.
Objects such as stars create what are known as continuum spectra. If we think about just the visible-light portion of the electromagnetic spectrum, a continuum spectrum looks like an unbroken rainbow of colours from violet at one end to red at the other.
Yet when we look at stars within this continuum we see dark lines, like those that Fraunhofer saw in the spectrum of the Sun. These are absorption lines.
They’re made when photons of light passing through the star’s atmosphere interact with electrons in the gases there.
These electrons can absorb the photons’ energy, removing certain wavelengths from the continuum. Which ones are removed depends on the atoms or molecules present.
By identifying the dark lines in a spectrum astronomers can determine the composition of the star’s atmosphere.
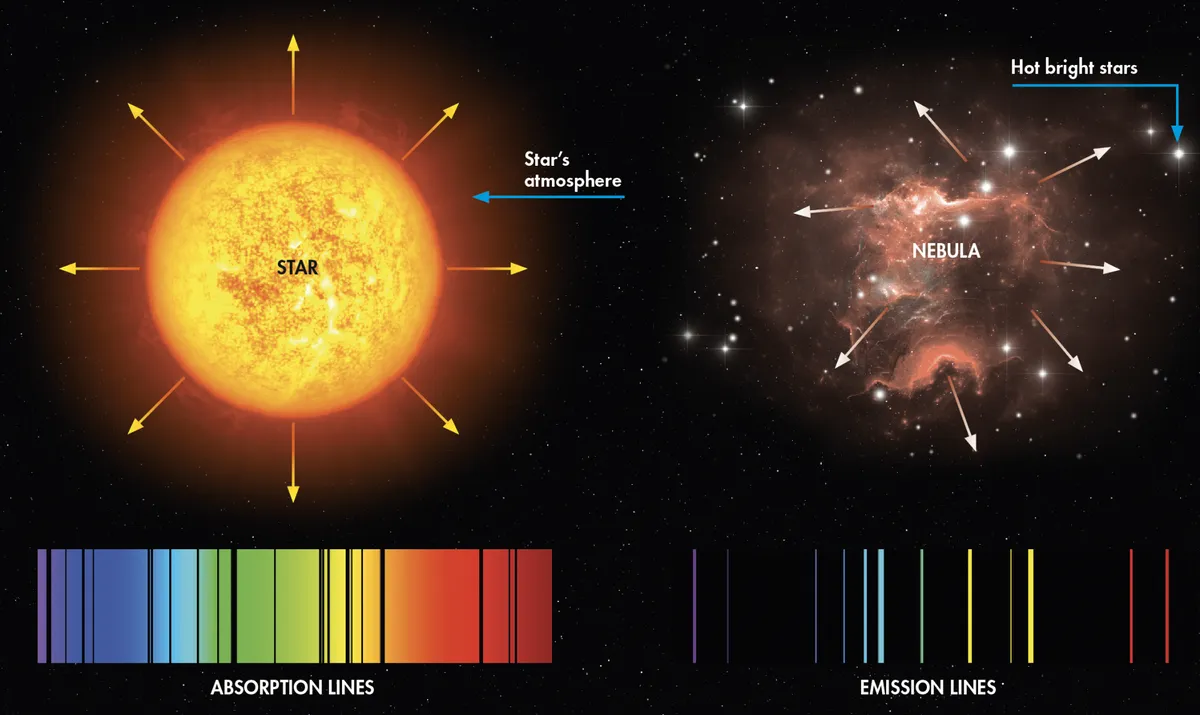
In emission lines you see the opposite. For example, photons from a bright star may be causing a nearby nebula to glow.
When this happens, the photons from the star give energy to, or ‘excite’, the electrons in the nebula’s gases.
Those electrons will eventually settle down and they do this by releasing energy in the form of a photon of light.
The wavelengths of the photons emitted are indicative of the atom or molecule that is glowing.
If you could observe the light from the nebula with a spectroscope, you’d see a series of thin, bright lines of different colours – these are emission lines.
Two centuries after Fraunhofer’s studies, astronomers are readying a new generation of spectrographs to explore the Universe, the likes of which have never been seen before.
Under the bright lights of the cavernous High Bay clean room at NASA’s Goddard Space Flight Center in Maryland, the James Webb Space Telescope is slowly taking shape.
Yellow scissor fences carefully partition off areas of the immaculate, white-floored room where instruments and other structures rest on substantial support stands.
High above, on an enormous gantry, each of the telescope’s 18 primary mirror segments sit protected within huge polished metal containers.
Gradually, the components are being meticulously tested and, eventually, integrated into the telescope’s skeleton, ahead of its planned launch in 2018.
Sitting in its own corner of the Goddard clean room, NIRSpec – the Near-Infrared Spectrograph – is just one of the four instruments that will fly on James Webb.
As with all of the telescope’s instruments, it will focus on observing the Universe at infrared wavelengths.

To understand why, you only need to look at the work of James Webb’s forebear, the Hubble Space Telescope.
Hubble has excelled at studying faraway galaxies as well as extrasolar planets and how they form within vast clouds of dust and gas.
But to peer further out into the Universe and deeper into nebulae you need to see the cosmos at much longer wavelengths, says NIRSpec instrument scientist James Muzerolle.
“Near-infrared and mid-infrared observations allow us to penetrate the dusty environments that are very important for studying star and planet formation,” he says.
Using NIRSpec, astronomers will also be able to study light from some of the most distant, and thus earliest, galaxies.
Light that has been stretched as it travelled across the expanding Universe. “The light that we’ll measure at our telescope will be light that was originally mostly in the ultraviolet [section of the spectrum] that’s been redshifted into the near-infrared,” explains Muzerolle.
“You have to be able to look at longer wavelengths in the near-infrared [region] in order to detect [the galaxies] at all.”

Like all spectrographs, NIRSpec will break down the light of celestial objects into spectra whose features, such as dark absorption lines, can tell astronomers much about what they’re looking at.
But NIRSpec’s great strength will be its ability to carry out what is known as multi-object spectroscopy.
“With a traditional spectrograph you have one slit that, generally speaking, is only big enough to put onto one object, like one star. You have to go one at a time to build up a large sample of spectroscopy of many different stars,” explains Muzerolle.
NIRSpec will be able to capture the spectra of around a hundred celestial objects, such as stars or faint galaxies, in one shot.
It’s able to do this thanks to an advanced piece of technology known as a micro-shutter array.
“Even on the ground there are not a lot of instruments that have used this kind of technology,” says Muzerolle.
In essence, the micro-shutter array’s role is to purposely block out large parts of NIRSpec’s field of view.

Light from the JWST’s 6.5m mirror will be fed into NIRSpec via a complex system of optics. But before it reaches the instrument’s grating, which spreads the light into a spectrum, it will first encounter the array.
Here a panel of roughly a quarter of a million microscopic doors, or ‘shutters’, can be programmed to remain either open or closed while the instrument is making its observations.
The result being that light will only be let through from a select few parts of the instrument’s field of view – the parts that contain the objects of which astronomers specifically want spectra.
“For example if you want to take spectroscopy of stars in a cluster you point the telescope at the cluster and then open the shutters at the positions of the stars that you’re interested in,” explains Muzerolle.
“All of the rest of the shutters [would be] closed so that you don’t get any background or other contaminating light getting through.”
The light going through the open shutters from the chosen targets will then fall onto one of NIRSpec’s gratings, which will produce spectra that can be imaged by the instrument’s extremely sensitive camera chips.
This image, of up to a hundred tiny infrared ‘rainbows’, will then be beamed back to Earth where it can be analysed by astronomers on the ground.
In capturing spectra of many stars at once, NIRSpec will allow astronomers to gather large samples of data that should shine a light on the processes at work in star and planet formation.
“From a statistical standpoint, this will really advance the field by a considerable amount,” says Muzerolle.
When the instrument surveys the most distant realms of the Universe it should also tell us more about how some of the first galaxies were born and how they evolved.
In doing so it will give astronomers a glimpse of the Universe as it was “a few hundred million years after the Big Bang”, says Muzerolle.
“We hope that we’ll be able to see many galaxies that are in the process of forming, of collecting their initial material,” he adds. NIRSpec isn’t the only spectrograph that will be operating on James Webb though.
MIRI, the Mid-Infrared Instrument, will study many of the objects that NIRSpec will. But because it observes in the longer wavelength, mid-infrared region of the electromagnetic spectrum it will also be able to examine some phenomena that NIRSpec can’t.
“With MIRI we’ll be able to study a whole range of astronomical objects from inside our own Solar System to the furthest reaches of the observable Universe,”says Sarah Kendrew, one of the astronomers who worked on MIRI.
“It is particularly sensitive to colder, dustier environments, the sort that we currently struggle to observe with the Hubble Space Telescope. Discs of dust around newly formed stars, for example, will be excellent targets for MIRI. As those are the birthplaces of planets, that’s a particularly exciting area of research.”
Spectrographs have been at the very heart of astronomy for two centuries. And one can only imagine the awe with which Fraunhofer might regard modern instruments, like MIRI and NIRSpec, if he could see them in their clean room today.
200 years ago his pioneering work revolutionised our understanding of the Universe.Now the legacy of that work is preparing to launch into space to start a new, and equally ground-breaking, revolution of its own.
Will Gater is an astronomy writer and journalist. This article originally appeared in the April 2014 issue of BBC Sky at Night Magazine.