We are living in the Sun’s extended atmosphere. Earth responds in the most magnificent way by producing beautiful curtains of light that dance across the northern and southern polar skies: the aurora borealis and aurora australis. But how does this incredible phenomenon occur and what drives it?
Well, that story begins with the Sun.
Rushing out of the Sun’s million-degree atmosphere is the solar wind; a stream of charged particles that blows throughout the Solar System, creating a vast bubble centred on our star.
Not even the immense gravitational pull of our star can hold back the solar wind and every second one million tonnes of the Sun’s atmosphere streams into space.
The incessant flow of the solar wind is only stopped when it collides with the interstellar medium, which lies beyond our Solar System.
- How to find the Northern Lights for yourself
- Best readers' images of the aurora
- How to detect the aurora with a homemade magnetometer
The solar wind is made mainly of hydrogen gas that has been ripped apart into its components of negatively charged electrons and positively charged protons, producing an electrically charged gas known as plasma.
As this plasma flows out from our star, it drags the Sun’s magnetic field with it. When the solar wind reaches Earth it is travelling at hundreds of kilometres per second and has a density of around 10 particles per cubic centimetre.
Compared to the 100 billion billion particles per cubic centimetre in the air around us, this density seems staggeringly small.
However, if you placed your fingertip in the flow of the solar wind you would have 500 million particles passing through it every second.

Earth’s cross-sectional area is one billion billion times bigger than this and so presents a much larger obstacle.
This raging torrent of particles isn’t able to bombard Earth directly. Instead it runs into an invisible shield – a vast magnetic bubble surrounding Earth that is created by currents flowing in the planet’s molten outer core: the magnetosphere.
Under most conditions the solar wind blows around the magnetosphere. However, if the magnetic field carried by the solar wind is pointing in the opposite direction to that of the magnetic field at the edge of the magnetosphere, a process known as magnetic reconnection can occur.
This joins the solar wind’s magnetic field to the magnetosphere’s outer layer, creating a channel for the solar wind particles to flow along.
Electrons trapped within the magnetosphere move backwards and forwards along the magnetic field lines.
But when reconnection occurs, they can be accelerated down toward the atmosphere in a process called precipitation.
The shape of Earth’s magnetic field funnels the electrons towards the magnetic poles – where the field lines converge and dive into Earth.
The fastest moving electrons make it to the top of the atmosphere where they collide with atmospheric gases, giving up some of their energy to the gas particles.
These gas particles then emit the energy they received in the form of light. Collectively, this produces the shimmering colours of the aurora at the edge of space.
The wavelength or colour of the light emitted in an auroral display depends on the type of atom that is energised and the energy exchanged in the collision.
Green light is produced by oxygen atoms at altitudes of around 100-150km, while red light is emitted by oxygen atoms higher up.
Blue and purple aurorae are produced by nitrogen atoms above 100km in altitude.
Aesthetically pleasing as these lights are, they are also a visual manifestation of the electrical currents that flow between the magnetosphere and the upper atmosphere.
Most of the time aurorae can seen as faint bands of light at high latitudes above northern Canada, Siberia and the Antarctic.
Ovals of auroral activity form around the north and south magnetic poles; their location reflects the base points of the magnetic field lines along which electrons are ‘precipitating’.
At times of high geomagnetic activity aurorae become much brighter, enhanced by the energy carried by fast moving solar wind streams, and
huge eruptions of the Sun’s magnetic field and charged particles – known as coronal mass ejections – which collide with the magnetosphere.
During these energetic events the auroral ovals spread in latitude so that bright displays can then be seen across Europe, possibly reaching
the south of England.
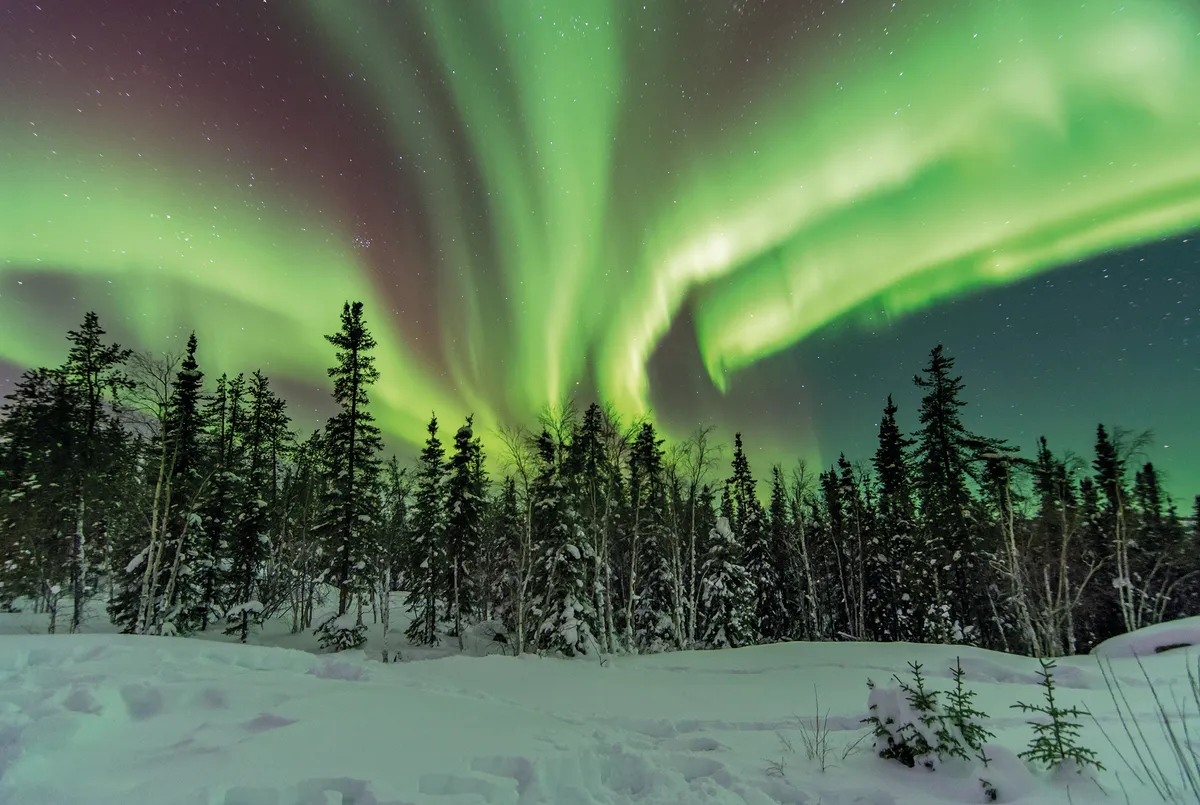
The frequency of auroral displays also shows a seasonal variation, with more being reported from March-April and September-October
This is a strange situation that seemingly implies that the Sun ‘knows’ about the position of Earth in its orbit!
This isn’t the case of course: it is the tilt of our planet – and therefore the magnetosphere – at these times that makes reconnection between the solar wind and Earth’s magnetic field more likely to occur, leading to an inflow of particles and enhanced currents.
Another explanation for this variation may lie in the light that the Sun emits – in particular the short wavelength ultraviolet light that is able to change the properties of Earth’s upper atmosphere by splitting the atoms into their electrically charged components, a process called ionisation.
How much of each hemisphere is illuminated varies as Earth moves around the Sun, meaning that the level of ionisation changes too.
This, in turn, alters how easily the currents that produce the aurorae can flow.
When a strong display does break out, undulating ribbons ripple across the sky, sometimes bursting into rays and arcs.
These dramatic shapes are thought to be produced by processes in the magnetosphere that cause the electrons to descend into our atmosphere, but the underlying physics is poorly understood at present.
Predicting a good aurora display in advance means forecasting when the Sun is going to emit a fast solar wind stream, or a coronal mass ejection, that carries a correctly aligned magnetic field capable of opening up the magnetosphere (if it reaches us).
This is not a trivial task and even the best models can only make predictions a few days in advance.
We do know that the strongest auroral displays are produced when Earth’s magnetosphere is severely disturbed by its interaction with the impacting solar wind or coronal mass ejection.
So, keeping an eye on ground-based measurements that indicate a disturbance in Earth’s magnetic field is the best way to decide whether you might be able to see the aurora and from where.
Websites like AuroraWatch UK and Space Weather are a good place to start.
The current state of the magnetosphere is measured on a global scale by what is known as the ‘Kp index’, a term first used in 1949.
The name originates from the German ‘planetarische Kennziffer’, which translates as ‘planetary index’.
The Kp index describes, on a scale of 0 to 9, how disturbed Earth’s magnetic field is.
The higher the value, the farther south the aurora tends to be seen.
As there are now many decades of observations it is possible to look up what Kp index is needed for you to see the aurora from your location.
Bearing in mind that aurorae do not need to be directly overhead to be seen – observers in the northern hemisphere with a clear view to the northern horizon and dark skies should look for them even if they are a few degrees in latitude away.

Observing and photographing the aurora
Seeing the aurora is a magical experience that deserves its status as one of those great natural phenomena everyone should try to spot at least once.
To the eye, most auroral displays – certainly the kind occasionally seen from the UK – appear as a green, perhaps pulsating glow.
Travel to the Arctic Circle, or witness a particularly strong display from the UK, and you may see more colours as well as many of the intricate structures, illustrated below, that make the aurora so beguiling.
If you want to take a picture of the Northern Lights, there are several things you’ll need to do to get the shot.
DSLR cameras are best for aurora photography as they allow you to open the shutter for long periods.
Some point and shoot cameras do have the ability to open their shutters for 30 seconds, but the images will likely be noisier than those taken with a DSLR.
To start imaging the aurora, first set your camera up on a tripod. This is crucial – you cannot hold a camera still enough to get a sharp shot for the length of the exposure needed.
Set your camera lens to its shortest focal length and open the iris of the lens right up.
Next, take some test exposures – with aurora photography you need to balance the length of exposure with the amount of detail captured.
Too long an exposure and the motion of the aurora will blur any fine structures in the display, too short and you won’t pick up anything at all.
Also vary the ISO sensitivity setting – if the display’s very bright you may find you can set the ISO to around 400 to reduce noise in your images.
Or, if you want to ‘freeze’ the display and capture fine structures like rays, try a shorter exposure with an ISO of 1600 or above, though the image may be noisier.
Prof Lucie Green is a Professor of Physics and a Royal Society University Research Fellow based at the Mullard Space Science Laboratory.