The rainbow of visible light we’re able to see with our own eyes makes up a tiny slice of a much broader continuum called the electromagnetic spectrum. Though you can’t see the rest, you’re probably familiar with many of them.
Beyond the red end of the visible spectrum is the infrared light detected by night-vision cameras, the microwaves you use to heat your dinner and the radio waves used in media broadcasts.
At the other end is the ultraviolet light that causes sunburn, the X-rays used in medical imaging and the gamma rays used in some cancer therapies. All except gamma rays are subdivided further, into smaller slices of the continuum.
Read more:
- What is a gamma ray burst?
- Splitting starlight: the science of spectroscopy
- What are magnetic fields and how do they shape the Universe?
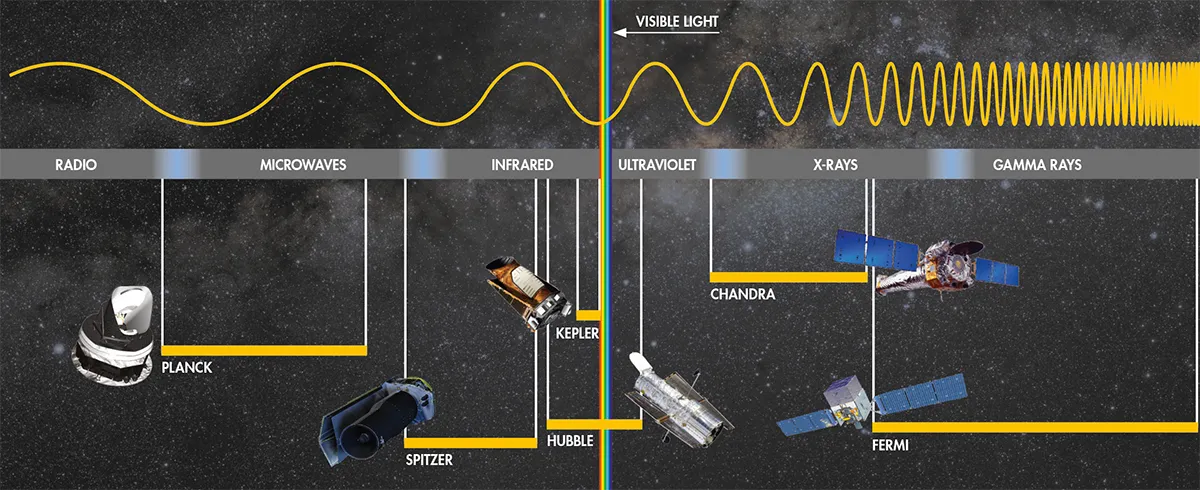
What is light?
All kinds of light are electromagnetic radiation, a form of energy made up of oscillating magnetic and electric fields, which spread like a wave.
The length of the wave determines the type of light, how energetic it is and how it interacts with matter.
Visible wavelengths are measured in nanometres (nm), a unit of length equal to one billionth of a metre.
The human eye can detect red light from about 700nm down to violet light at about 400nm, a very small section.
At the extremes, the lowest-energy radio waves have wavelengths measuring thousands of kilometres, while the most energetic gamma rays have wavelengths that are smaller than an atom at a few trillionths of a metre.
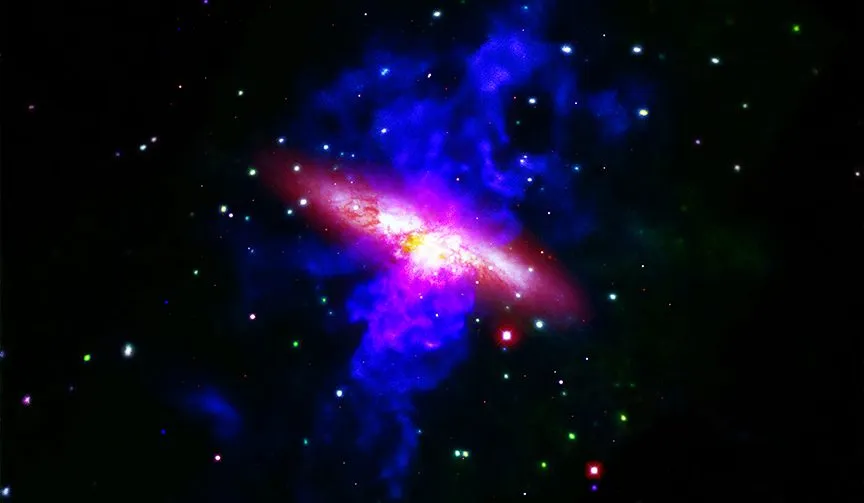
Invisible wavelengths
We can ‘see’ the non-visible wavelengths of the electromagnetic spectrum using dedicated professional telescopes.
Radio waves can be recorded using single dishes or arrays spread over large areas, but most other wavelengths are heavily absorbed by Earth’s atmosphere and must be observed from balloons, rockets or satellites.
Why bother? Because observing at multiple wavelengths provides astronomers with a more complete view of the cosmos.
In fact, many types of celestial object can only be seen in non-visible light. An optical telescope, no matter how large, would not be able to detect gamma-ray bursts, the most energetic explosions in the Universe – as their name implies, astronomers need a dedicated gamma-ray telescope.
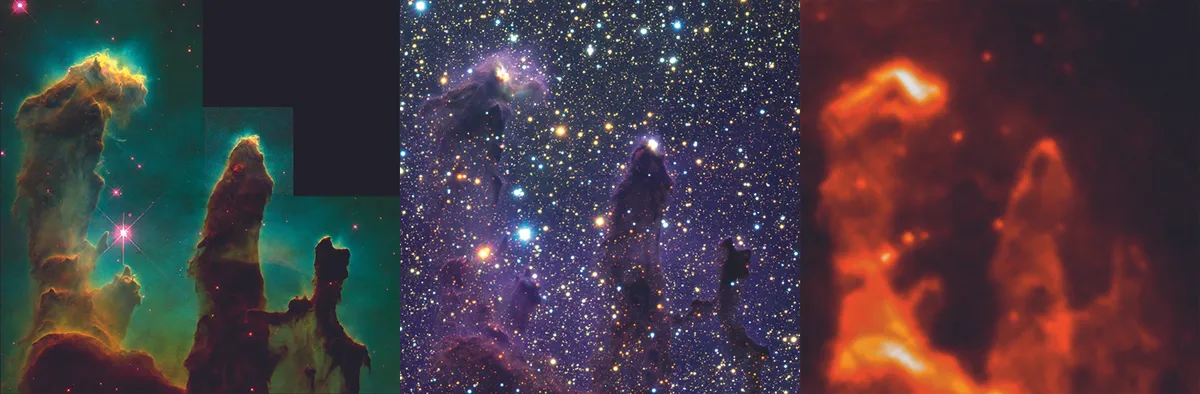
Likewise, radio telescopes are required to detect the jets and lobes fired hundreds of thousands of lightyears into space by the supermassive black holes at the centre of radio galaxies.
Even the objects we can see in the visible part of the spectrum benefit from further study. Clouds of cold dust and gas in the Milky Way and other galaxies block visible light, causing them to appear opaque, but in infrared light these stellar nurseries are near transparent, revealing the young stars forming within.

On a larger scale, we can peer deeper into galaxy clusters: as well as using visible telescopes to see the galaxies themselves, astronomers can map the intra-cluster gas using X-rays and reveal the black-hole driven jets capable of excavating huge bubbles in this gas using radio waves.
Exploring the electromagnetic spectrum has even helped us to understand the Universe a bit better. Data from ESA’s microwave-focused Planck satellite has produced the most detailed map of the cosmic microwave background, the afterglow of the Big Bang.
And in cosmological redshift – where the light from a deep-sky object increases in wavelength so it ‘shifts’ from its anticipated position towards the red end of the spectrum – we have one of the strongest pieces of evidence for the idea of an expanding Universe.
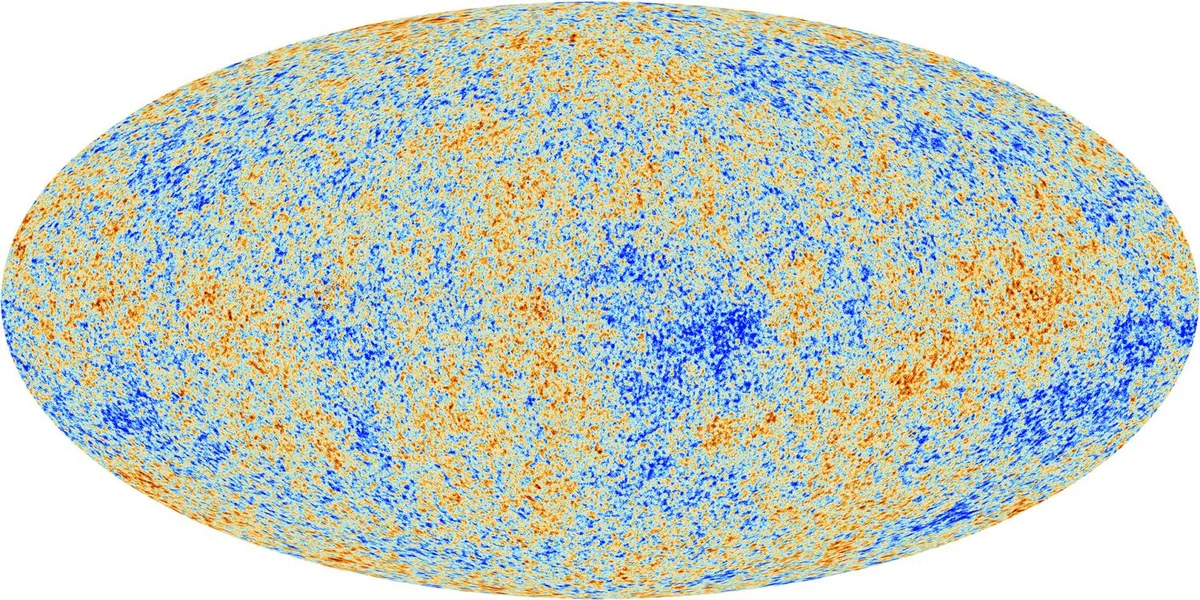
Using the visible spectrum
The visible part of the spectrum is small, but not insignificant. There is still a lot to see.
One simple way you can make the most of this narrow slice is by controlling which wavelengths you see using filters. For example, light pollution filters transmit a broad band of wavelengths, but block the ones commonly produced by streetlights to give you a clearer view.
Filters with narrower wave bands may only let through certain colours of light, which can be useful to increase contrast or enhance specific features on planets or in nebulae.
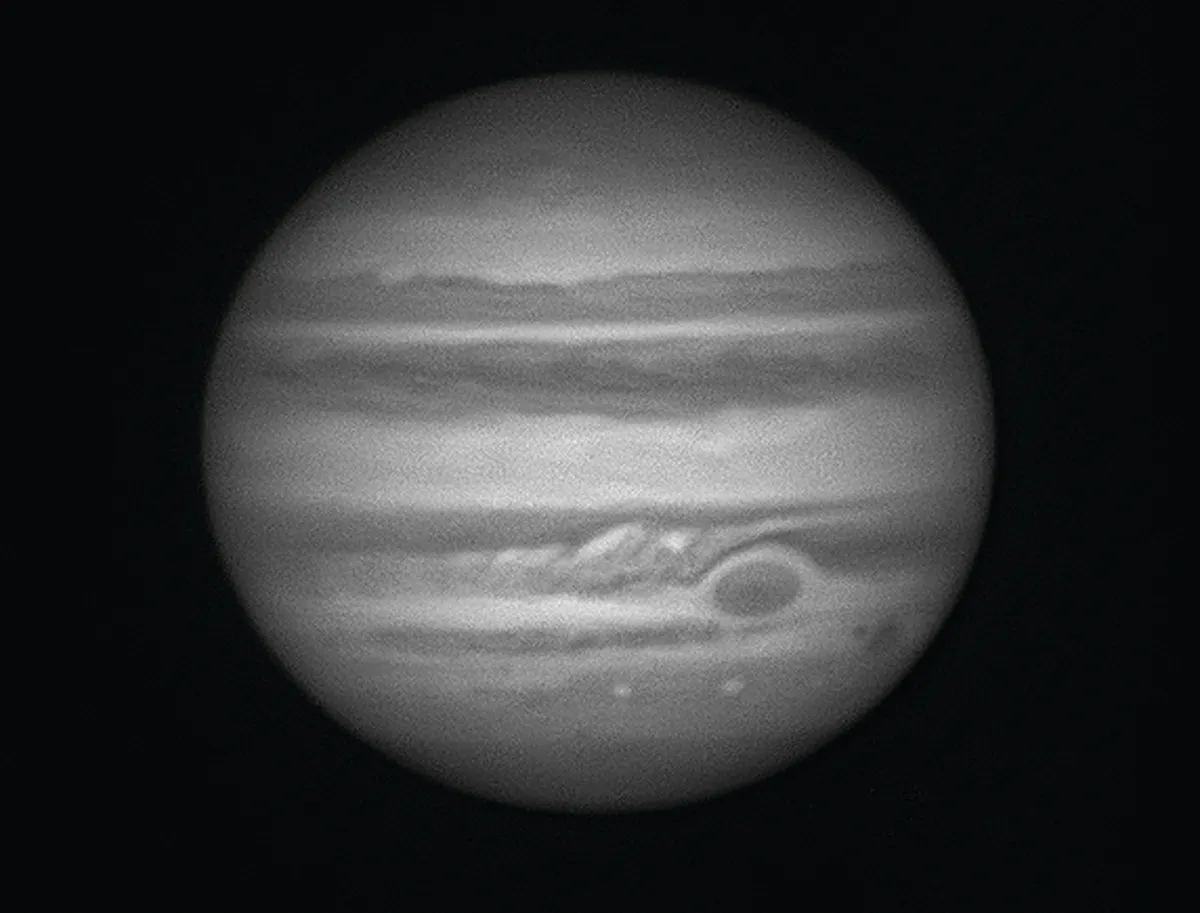
Viewing Jupiter through a filter that only transmits blue light makes its red features, such as the Great Red Spot, stand out as dark shapes against a bright background.
Even more precise filters are used to isolate individual wavelengths emitted by specific atoms, such as hydrogen, oxygen or silicon, and are particularly useful for observing emission nebulae.
When it comes to the Sun, filters tuned to the deep-red wavelength of hydrogen alpha block out the brilliant photosphere to reveal solar prominences and other features in the chromosphere beneath.
This guide originally appeared in the May 2013 issue of BBC Sky at Night Magazine.