What have been the biggest discoveries in the history of astronomy and space science?
Galileo’s telescope, Newton’s apple, Einstein’s equation? History is filled with critical moments when scientific endeavour took a huge leap forward, and space and astronomy are no exceptions.
Ersilia Vaudo is a space scientist at the European Space Agency whose book, The Story of Astrophysics in Five Revolutions, explores the biggest ‘eureka!’ moments in astronomy, when our understanding of the Universe was changed forever.
More amazing space

Here, Ersilia gives us her top five astronomical epochs, and reveals how these great discoveries revolutionised our ideas about the cosmos, the future and the very nature of existence.
SQUIRREL_13209713
Gravity governs the Universe
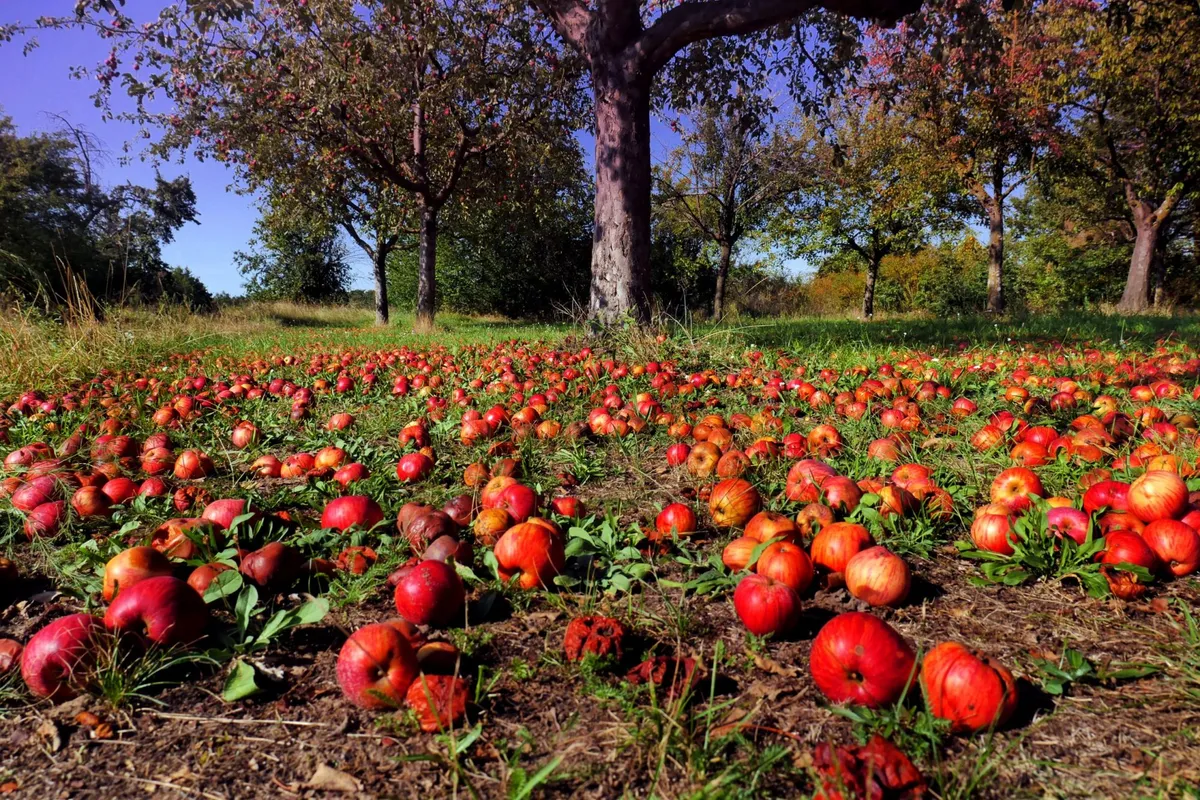
Newton's law stated that attraction between bodies depends solely on mass and distance. Yet he couldn't explain how bodies millions of kilometres apart influence each other's motion.
Newton posed this question in his Principia, and he left it unresolved.
About 200 years later, Einstein pondered a point. There is a distance of 93 million miles (150 million kilometres) between the Sun and Earth.
This space, which until now had been considered nothing more than a passive background, may in fact play a role in the dynamics of their mutual attraction.
Einstein transformed this understanding with a revolutionary leap: spacetime itself is the gravitational field.
Imagine spacetime as a rubber membrane, curved by the weight of celestial bodies. The fabric deforms, and this shape determines how objects move along it.

Gravity becomes a geometry impressed in spacetime rather than a mysterious action at a distance.
In general relativity, gravitational attraction is simply the effect of spacetime curvature created by mass.
Earth orbits the Sun because it follows a natural trajectory in curved spacetime, rolling along a valley created by our star's mass.
The nature of gravity is revealed. No longer a force that pulls and holds a body that wants to flee, but a line emerging from the curvature of spacetime, a path within which objects glide effortlessly.
The Universe becomes a soft and dynamic landscape, filled with valleys and dips, curved lines in which objects move fluidly, no longer buffeted by the pull and push of gravity.
The roles change. Her Majesty, Gravity, is transformed into a sinuous design woven by the hand of matter and energy into the elastic fabric of the Universe.
Space and time are bound together
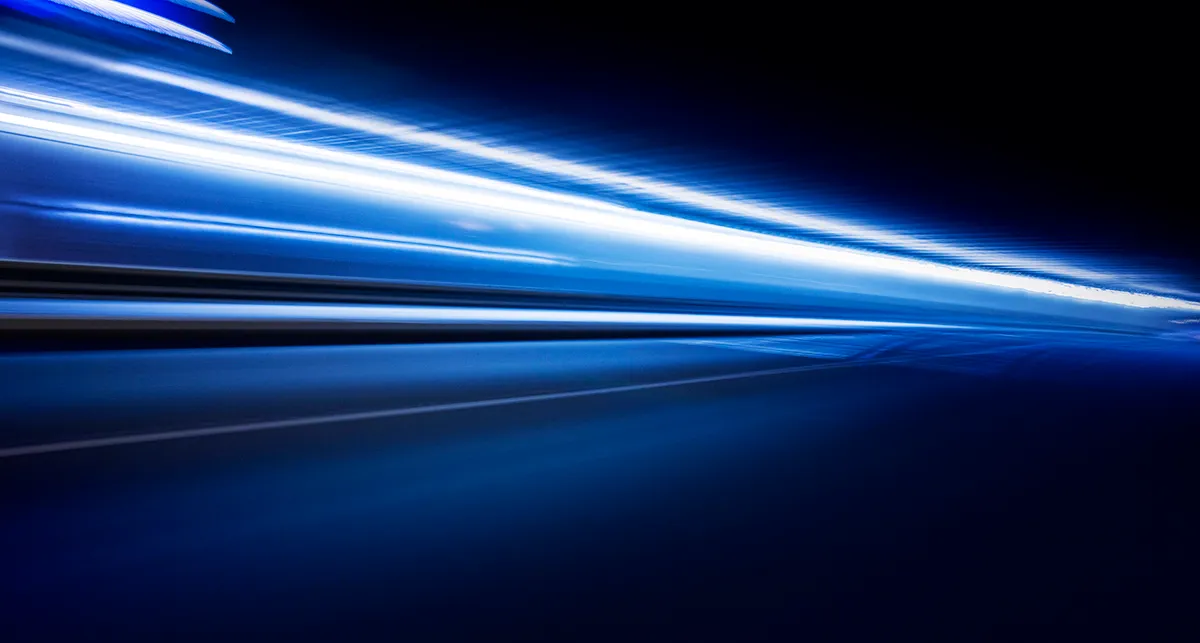
The upheaval of the physical world as it was known began with this realisation: the speed of light is a constant that does not change when moving from one frame of reference to another.
As we know, speed is calculated from the distance travelled and the time taken to travel it, i.e. v = s / t.
Newton’s vision of absolute time and space was only compatible with a speed of light that assumes different values for observers in motion.
Space and time are absolute, and velocity is relative, depending on the motion of the observer.
Once the speed of light is fixed, and independent of the motion of observers, space and time must be able to continuously ‘adjust’ each other so that their ratio remains constant and always equates to the speed of light for any observer.
In other words, once the speed of light has been established as a fixed and insuperable limit, space and time pay the consequences.
They must start to adjust to each other in order to keep the speed of light constant in any reference system.
They lose their comfortable Newtonian absoluteness. Bound to the speed of light by an arithmetical division, they are forever, inextricably linked, and their true inner physical nature emerges.
The concept of spacetime comes into play, and time, alongside the spatial triad, becomes the fourth dimension.
Gravity pulls time down like an apple

The geometry of the Universe, its inherent structure, is a dynamic entity that deforms, ripples, twists and in some places sinks. It all depends on the energy and matter that fills it.
To quote a famous phrase attributed to the American physicist John Wheeler: "Spacetime tells matter how to move; matter tells spacetime how to curve."
Right up until the first direct measurement of gravitational waves in 2015, much of what we knew about the Universe, at least from a certain point in its evolution onward, was taught to us by light, or its absence.
But gravity often intervened to complete the story, luring out what sought to hide, flushing out the unseen presences. Unearthing black holes.
A distinctive imprint of a black hole in the soft tissue of spacetime is the event horizon.
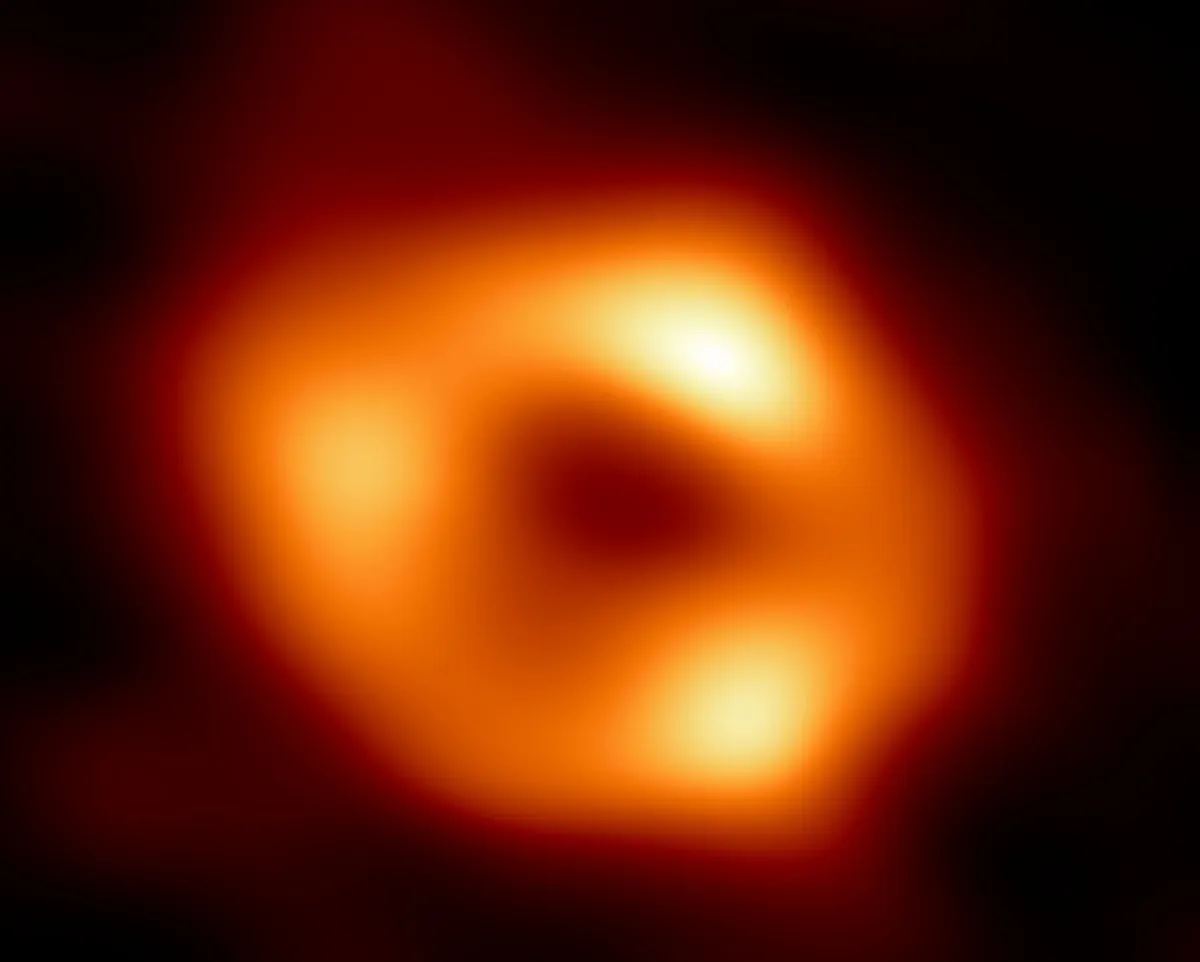
It is a terrifying threshold, a line of no return that marks the entrance to the vortex of spacetime, created by the presence of an enormous amount of mass concentrated in a very small space.
It is the limit beyond which nothing, whether matter or radiation, can escape.
In a black hole, the immense gravitational force, which is billions of times greater than that of the Earth, traps information and light, and the extreme deformation of the spacetime curve causes an extraordinary slowing of the flow of time.
If we were to spend one year in orbit at a hundred metres away from the event horizon of the black hole Sagittarius A*, which lies at the heart of our Galaxy and has a mass four million times greater than that of the Sun, 11,000 years would have passed on Earth in the meantime.
The expansion of the Universe

Who could have imagined the Universe had a beginning?
Even Einstein himself resisted this implication emerging from the equations of his 1915 general relativity theory by introducing a 'cosmological constant' to preserve a static universe.
The mathematical breakthrough came from Alexander Friedmann in 1922. Working with Einstein's equations, he demonstrated that the Universe could be expanding or contracting.
Independently, Georges Lemaître reached similar conclusions in 1927, proposing that an expanding universe implied a beginning point: what would later be called the Big Bang.
The decisive evidence arrived through Edwin Hubble's work at Mount Wilson Observatory.
Using the 100-inch Hooker Telescope, Hubble first confirmed in 1924 that spiral ‘nebulae’ were really distant galaxies far beyond our Milky Way.
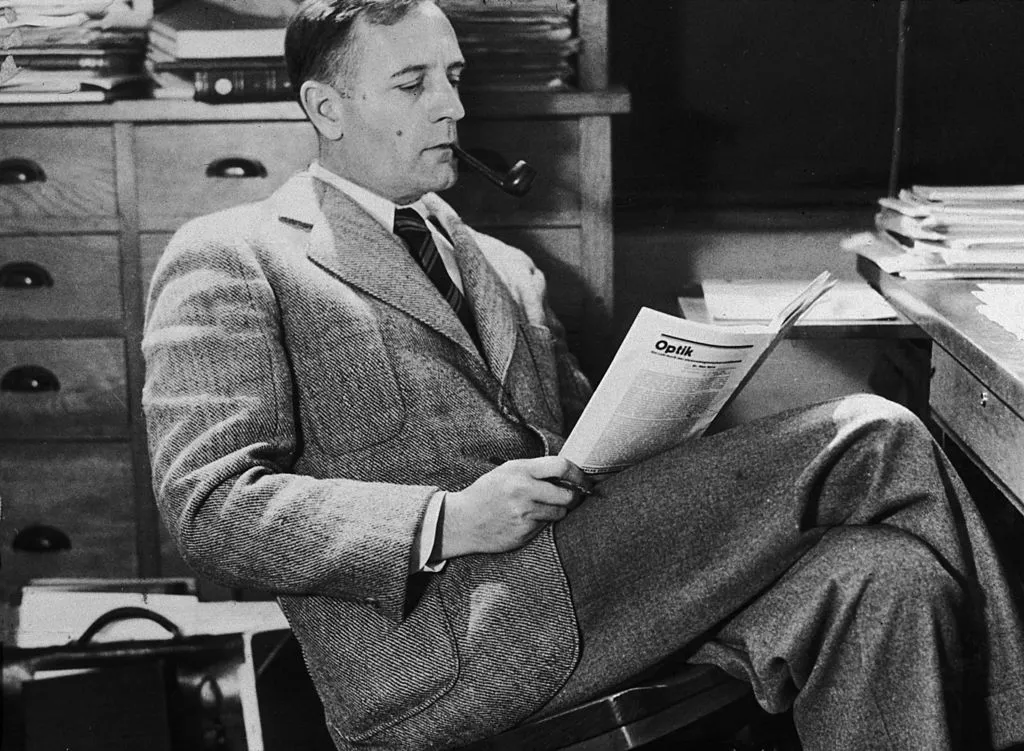
Then in 1929, combining Slipher's redshift data with his own distance measurements, Hubble discovered a pattern: more distant galaxies moved away faster than closer ones.
This relationship – now known as Hubble's Law – provided compelling evidence for cosmic expansion.
When Einstein learned of Hubble's findings, he abandoned his cosmological constant, allegedly calling it his "greatest blunder" (though this quote may be apocryphal).
The expanding universe theory gained further validation in 1965 when Arno Penzias and Robert Wilson accidentally detected Cosmic Microwave Background radiation – the afterglow of the Big Bang – cementing our understanding of a universe born from a singular point, expanding ever since.
This discovery transformed our cosmic understanding.
It indicated a universe with a beginning (the Big Bang 13.8 billion years ago) rather than an eternal, static cosmos.
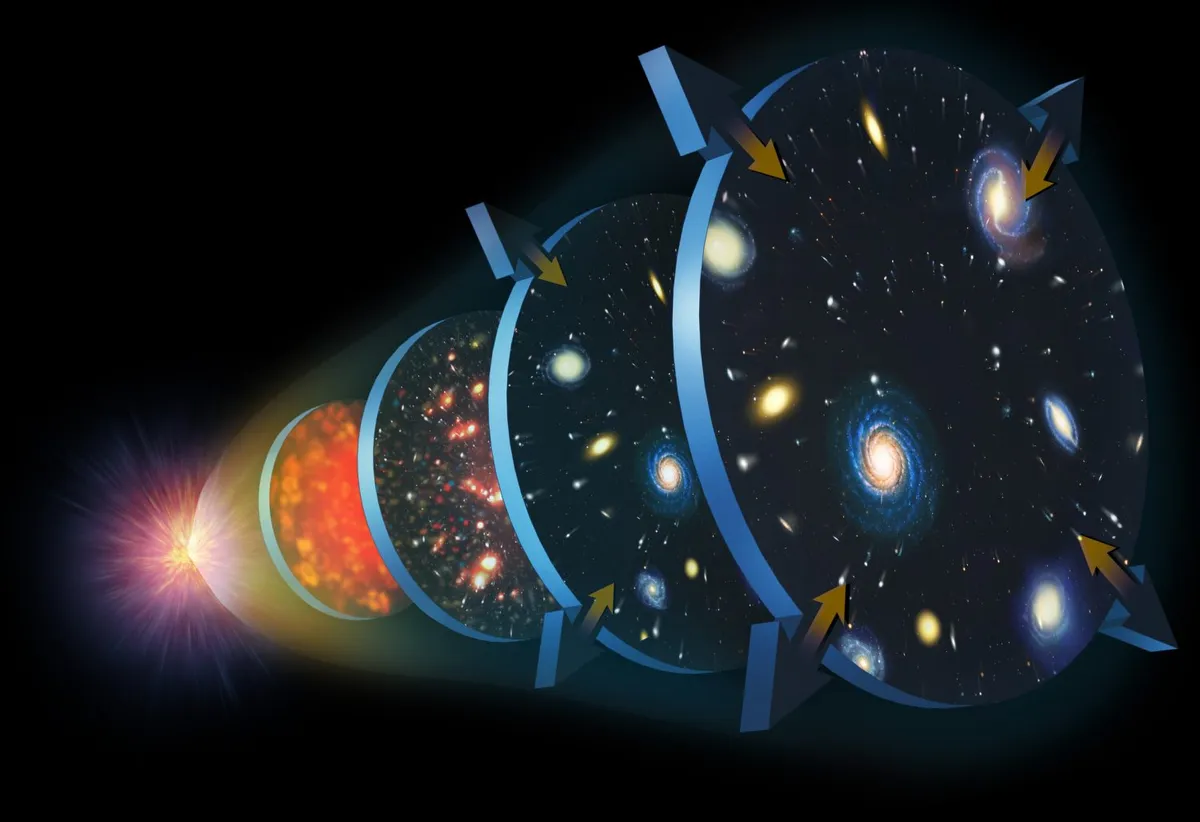
It also suggested possible futures: eventual collapse, balanced state, or – as current evidence indicates – accelerating expansion toward a ‘Heat Death’ where galaxies drift eternally apart.
Beyond astronomy, cosmic expansion changed philosophy by placing humanity within a universe that has both a beginning and an end.
It raised profound questions about what caused the Big Bang, what exists beyond our observable Universe and whether multiple universes exist.
A single discovery thus revolutionised not just our view of the cosmos but our understanding of existence itself.
There's something rather than nothing

In the primordial moments after the Big Bang, the Universe existed in a state of unimaginable heat and density.
Within this cosmic cauldron, the same amount of particles of matter and antimatter, spontaneously formed, collided and annihilated each other in a perfect symmetrical dance of creation and destruction.
According to our understanding of particle physics, these opposing forms should have been created in equal amounts, leading to their complete mutual annihilation.
Yet here we are, a Universe composed of stars, planets and galaxies, all made of matter.
Something profound happened in those first fractions of a second of cosmic history.
A subtle imbalance emerged, a whisper of asymmetry in the laws of physics that allowed matter to gain the slightest advantage over antimatter.
This asymmetry, though nearly imperceptible, had monumental consequences.

The magnitude of this imbalance staggers the imagination.
For every ten billion pairs of particles and antiparticles that formed and subsequently annihilated each other, just a single particle of matter survived.
This minute surplus – one part in ten billion – constitutes everything we see in the observable Universe today: every galaxy, star, planet and living organism.
As the French astrophysicist Michel Cassé poetically expressed: “In the beginning, there is genesis, but there is also murder. Half the sky vanishes.” ("Au début, il y a genèse, mais il y a aussi meurtre. La moitié du ciel qui disparaît.")
This asymmetry represents one of the greatest unsolved mysteries in physics. And the reason why we are here asking this question.
"Why is there something rather than nothing?" Leibniz asked himself in Principles of Nature and of Grace Founded in Reason, adding that 'nothing' would have been a much simpler solution after all.
A complex philosophical question, and the most charged according to Umberto Eco.
This inexorable tension to understand what we do not know, asking questions and strive for answers, is also what make us part of that extraordinary 'something'.
- The Story of Astrophysics in Five Revolutions, by Ersilia Vaudo, is published by W.W. Norton & Company.