Chris Impey is an astrophysicist based at the University of Arizona in the US.His latest book, Einstein's Monsters, is a look at the physics behind black holes and their role in the functioning of the Universe.BBC Sky at Night Magazine spoke to Impey to find out more about these dense objects, from which not even light can escape.
Read an excerpt from his book on the discovery of the first black hole below the interview.
Do you think black holes are generally misunderstood by most people?
Yes, on several levels! To truly understand them you need to know general relativity, and that’s a tiny fraction of the population.
The number of people in the world who have what you might call a deep physical understanding of it is probably just a few hundred.
But at the other extreme is what pop culture has told us about black holes, which is that they are the Hoovers of the Universe, vacuuming up everything, when in fact all the effects of relativity and the extreme gravity happen pretty close to the event horizon.
Black holes are also statistically very rare, so the nearest examples are hundreds or even thousands of lightyears away, and you’d have to be really close to them for anything bad to happen to you.
If you’re making a little worry list because you’re voyaging out and exploring the Universe, I’d say black holes would be way down there on your list of concerns.
But black holes are not fully understood in terms of conventional physics, so there are still mysteries to be solved because we haven’t recognised the quantum scale understanding of a black hole with the macroscopic gravity theory.
That’s a tension in the subject that affects the research.
I think it’s interesting to people when they find out that black holes are like testing grounds for theories: we don’t know about the information in a black hole, or what happens to it.
You could break one of your fundamental theories just by carrying out good enough observations of black holes.
Is there a succinct way of describing a black hole and how they form?
The conventional understanding of black hole is that, in a star’s life, there is an eternal battle between gravity pulling inwards and pressure from nuclear reactions and fusion pushing outwards.
At the end of a star’s life, it will always run out of nuclear fuel and gravity will always win.
If the star’s core is about three times the mass of the Sun, then according to our best theories it will collapse to the point where you end up with a black hole because there is nothing to resist the compression.
There is an imaginary surface called the ‘event horizon’ where the escape velocity is the speed of light, and therefore nothing can escape it.
The event horizon itself is probably another misunderstanding!
It’s a mathematical surface; it’s not really a physical barrier or boundary or anything like that.
It separates the part of the Universe where we can learn things or shine radiation and talk to each other, and the part where that’s all trapped and we don’t know what goes on.
Since the 1930s that’s been considered to be the end state of massive stars, and it took decades to find even a dozen good examples, so it’s been pretty slow progress.
I think the more exciting and less well-known part is the idea that every galaxy has a black hole at its centre and they’re huge.
Our Galaxy, being a fairly modest-sized galaxy in comparison to a giant elliptical, has a pretty modest black hole four million times the mass of the Sun.
But they can reach up to a thousand times bigger than that, and that’s incredible.
So you have these insanely big and massive black holes at the centres of galaxies ranging up to about 20 billion times the mass of the Sun.
While you would have to squash the Sun to a size 3km across in order to make it a black hole, the event horizon of the biggest black hole is the size of the entire Solar System.
Can we conclude that black holes play an important part in the formation of galaxies, or do these black holes form later once the galaxy is established?
It’s sort of a chicken-egg situation that hasn’t been resolved.
Partly because we can’t observationally access the true formation era of galaxies.
The James Webb Space Telescope has been designed to see back to the first light of the first galaxies forming, but we’re going to have to wait a few more years for its launch.
In the meantime, since we don’t have observations that go within the first few hundred million years after the Big Bang, then the theorists are speculating.
Some say that out of the early, hot dense Universe you could get gas collapsing to form ‘seed’ black holes more massive than the stellar black holes we have now, which are a few tens times the mass of the Sun.
In the early Universe they actually could be a few hundred times the mass of the Sun.
In computer simulations we can form these seed black holes and they would nucleate the first small galaxies and everything grows by merging: when galaxies merge their stars combine and the black holes combine and everything just grows.
I think that’s still the favourite idea, but you could also say that the first small galaxies formed really early and then within them, stars collapsed to the centres and formed black holes.

Black holes were theorised long before they were actually confirmed, weren’t they?
They were theorised well before Einstein.
John Michel was an English clergyman and in the late 18th century he speculated about black holes.
He was just extrapolating from Newton’s theory of gravity.
If you have a massive object with an escape velocity, what if you make that object even bigger and more massive?
Eventually the escape velocity equals the speed of light.
It turns out, in fact, that nature makes them by having a very dense object rather than just having a very large, massive object. But it was a sensible speculation.
Eventually the idea lapsed and people considered it to be a quirky idea and that there would be no way to observe such a thing, because how could you observe a dark star?
They then properly emerged out of general relativity, and Einstein himself didn’t play a huge role in the theory of black holes early on because he thought they were an artefact; he thought they were not real.
Because of that, he missed some of the predictions from his own theory that were confirmed decades later, like gravitational lensing.
In several ways he didn’t take his own theory seriously enough!
It was left for people a bit later on in the 1930s, like Oppenheimer.
When he was working on the bomb, basically, he was essentially looking at nuclear matter states that were getting close to black hole states, and so out of those calculations that you would do to work out how a bomb works, he realised there was probably a density at which nothing can resist the force of gravity and that the end point of that collapse is what we would call a black hole.
He and other physicists in the 1930s pretty much nailed down the idea of a black hole but again it was just set aside, because how could you possibly observe that?
In 1969 the first black hole was nailed down: Cygnus X-1 and the X-rays being given off were the key.
We know that matter falling into a black hole can create energy to blast jets outwards.
But how is that matter able to escape the black hole?
The key to how black holes are able to pull matter in but also radiate a lot of energy and accelerate particles is the fact that they are spinning.
A more massive star than the Sun, by the time it’s collapsed to black hole-size, it’s spinning hundreds or thousands of times a minute.
Matter falling towards it from any direction will eventually settle into a disc.
It’s pretty standard physics that if you have a small, spinning gravity object that you would have a disc of material around it.
The gas settles into an accretion disk and gets very hot because it’s moving fast and the gas particles are bashing into each other, heating up to tens of thousands of degrees, and so the accretion disk is going to shine in ultraviolet or X-rays.
This is how we find black holes. When you observe the X-ray sources of a black hole, you are really seeing the accretion disk.
The matter is actually going into the black hole rather inefficiently; a black hole doesn’t actually eat very much.
The big black holes at the centres of galaxies really consume about one solar mass a year; so that’s the equivalent mass of our Sun every year.
They’re hundreds of millions times more massive than the Sun, so that’s a pretty slow rate.
The gas only falls into the inner boundary of the black hole’s accretion disk rather slowly, and because of the angle and the momentum of the black hole there are escape channels and acceleration channels along the poles.
The plasma - very hot gas - is very slowly funnelling in through the accretion disk, and it’s accelerating very fast along the poles, where it can escape.
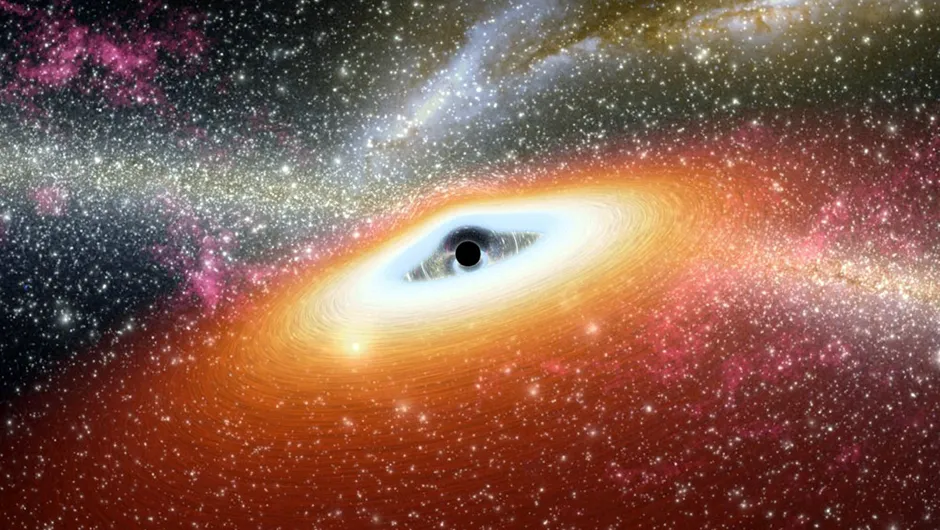
Are there any types of black hole that we’ve yet to discover?
We’ve found everything from black holes a few times the mass of the Sun all the way up to 20 billion times the mass: the record holder.
There’s a gap in the middle, between the mass of the Milky Way, which is a few million times the mass of the Sun, and the biggest stars, which might be a few hundred times the mass of the Sun.
There’s a big research effort to find these things, because they’re rare.
It would be difficult to find a 100-solar mass or 10,000-solar mass.
You can find them either in the nuclei of small galaxies or in large globular clusters, which are the largest star clusters in the Galaxy.
One thing that’s hypothesised but not yet observed is microscopic black holes.
They are sometimes called primordial black holes because according to the theory of the Big Bang there are stages of the early Universe when the density and temperature are extremely high, when gravity is strong enough that it could in principle form microscopic black holes, say a kilogram or the mass of a person, or even the mass of a planet.
Some people even wonder whether a large collection of these microscopic black holes could actually be dark matter.
If Hawking’s theory is right and black holes evaporate, these little ones would evaporate very quickly.
So there’s a sort of trade-off in the early Universe between the formation of these small black holes and the end of their formation, because the Universe is expanding and so eventually cannot form them, plus the fact that black holes evaporate eventually and the small ones evaporate faster.
There’s a tricky calculation as to whether any of these small black holes could survive in the present day, and what mass they would have.
It’s a controversial calculation because we just don’t know enough to be able to get it right.
In the book you discuss the possibility that an alien civilisation might be able to harness the energy of a black hole.
How would a civilisation do that, and what might they be able to do with it?
The thing about black holes is that, when they’re accreting material they are incredibly efficient radiators of energy.
So these luminous distant galaxies are caused by supermassive black holes that are accreting material and pumping out a thousand times the energy of an entire galaxy.
They are one of the most efficient energy sources conceivable in physics, so if you were a civilisation that wanted to harness a lot of energy, you might be able to find some way of harnessing the energy of a spinning black hole.
It’s about 100 times better than using the light of your host star, which is what we do!
Another speculation is if you were in the realm of the future Universe and all the stars have died, you don’t have any starlight as energy, then your only energy source is gravitational energy.
If you wanted to fuel a civilisation of the very far future a trillion years from now and your only energy source is gravity, then a black hole is one of the most efficient places to look.
In the super far-distant future, you have an extra option due to Hawking radiation.
If Hawking was right, then black holes are also radiating a very small amount of energy.
In this distant future, they won’t be accreting any matter, so they’re really just a spinning black hole in space.
So you would have to harness either their spinning energy, or their feeble radiation.
If there’s any kind of sentient life that far in the future and they want to fuel their activity, that’s pretty much what they’re going to have to do!
Are you also saying that black holes will be the last objects to exist in the Universe?
Pretty much! The physics theories that say normal matter is unstable and protons eventually decay, also say that this will happen long before the decay of black holes.
Since black holes have sequestered whatever went into them in a sealed-off piece of spacetime, in that sense they will be the last objects in the Universe.
There will be particles in that far future: neutrinos, electrons, anti-neutrinos and positrons, but that’s probably it. And there will be black holes!
Every form of normal matter that isn’t a black hole will decay, and so they’re the go-to objects of the very far future.
The biggest black hole - the 20 million solar mass black hole - its evaporation timescale is of an order 10100 or 10105 years.
This is the longest timescale you can sensibly talk about in astrophysics.
So the civilisation of the far future would have to go to the centre of what had once been a large galaxy, where all the stars have died and evaporated into intergalactic space, and there’s a huge black hole that’s slowly evaporating, but it’s not accreting anything because there’s nothing to accrete, they would have to hunker down in that corner of the Universe.
But personally I think we have more proximate concerns!
An excerpt from Einstein's Monsters: Finding the First Black Hole
It was 1964. The Beatles had taken America by storm and a brash young fighter called Cassius Clay had become heavyweight champion of the world. Science was booming as well.
The term 'black hole' appeared in print for the first time in January of 1964, and in June of that year a small sounding rocket launched from New Mexico identified a strong source of X-rays in the constellation of Cygnus, the swan.
'Black swan' is the term for those rare, unexpected events that play an outsized role in the development of science.
It’s also used by philosophers to talk about the problem of induction, where seeing many white swans isn’t evidence that black swans don’t exist.
Finding the first example of a 'black swan' in physics involved seven years of detective work.
X-ray astronomy was a new field in the 1960s.
High-energy radiation from cosmic sources can only be detected from space; the first source had been found two years earlier.
The eight sources from the 1964 observations were consistent with supernova remnants, or hot gas created when a massive star dies violently.
The discovery observations had poor spatial resolution so didn’t narrow down the location of the X-rays emanating from Cygnus to a region much smaller than the constellation itself.
In 1970, the Uhuru X-ray satellite showed that the intensity of Cygnus X-1 varied in less than a second.
Astrophysicists use time as a way to measure the size of remote objects; the idea is that intensity changes can only occur in the time it takes light to travel across the source.
The intensity variations on Cygnus X-1 suggested that the object could be no larger than 100,000 kilometers across, a tenth the size of the Sun.
Further progress required radio astronomy.
An accurate position on the sky from the National Radio Astronomy Observatory identified the variable X-ray source with a blue supergiant star called HDE 226868.
Supergiants are hot stars but they’re incapable of emitting copious amounts of X-rays.
Something in that region of space was heating gas to a temperature of millions of degrees.
The decisive next step used optical techniques.
In 1971, two groups took spectra of the blue supergiant and found periodic variations in the Doppler shift of the star that matched the variations in the X-ray emission.
Orbital calculations allowed the researchers to estimate the mass of the “invisible” companion that was tugging the supergiant.
The researchers speculated that a black hole was sucking gas off a companion star and that this gas was somehow heated enough to a temperature high enough to produce X-rays.
As astronomer Tom Bolton prepared to present a paper on these findings at the American Astronomical Society meeting in Puerto Rico, he was wracked with nerves.
He was only 28 at the time. “Five minutes before I gave my paper I was revising it on the fly.
I was sitting at the back of the room trying to get the latest data in my diagram,” he recalled.
He was also feeling of the pressure of competition.
He was just one year out of his Ph.D. and working alone.
A more experienced team at Royal Greenwich Observatory was using a larger telescope to get similar data on Cygnus X-1.
Everyone was cautious since careers had foundered on false claims of detecting a black hole.
Within a year, Bolton was sure and he staked his reputation on it.
He presented the research at the Institute for Advanced Study in Princeton—the academic home to Einstein and Oppenheimer.
The observations were robust.
The first black swan had been found.
By the late 1970s, black holes had entered the popular culture.
Their bizarre properties fascinated people who rarely gave a thought to astronomy.
Disney released a movie called 'The Black Hole' and its ominous theme earned it a PG rating, a first for Disney.
Although the movie is low-tech and cheesy in parts, it was ambitious for its time and it presented black holes as metaphors for death and transfiguration.
The pop innocence of the Beatles evolved into raucous rock. Rush, Queen and Pink Floyd all gave nods to astrophysics.
Let's take a closer look at the archetype, Cygnus X-1.
From the Earth, we look towards the constellation of Cygnus, riding high in the summer sky.
We home in on a region near the center of the cross marking the body of the swan.
With good binoculars we can see a blue-white star nestled in a loose group of hot, young stars that all formed at the same time.
They shine like diamonds scattered onto black velvet.
Five million years ago, when our primate ancestors split off the evolutionary tree, these stars congealed from a collapsing cloud of gas and dust.
The blue-white star of interest is 6,000 lightyears away, near the edge of a neighboring spiral arm in the Milky Way.
That’s a prodigious distance of 20 thousand trillion miles.
For it to be visible so easily the star must be extremely luminous, emitting four hundred thousand times more energy than the Sun.
The light is old. It left the star when there were less than a million humans on the planet and the first pharaohs of Egypt were sitting on the throne.
We approach our prey cautiously.
At the distance of the Earth from the Sun, the star would be blindingly bright, twenty times the size of the Sun - the width of two spread hands held at arm’s length.
This blue supergiant is locked in a 6-day orbit with a near-invisible companion closer to it than Mercury is to the Sun.
But the companion isn’t totally dark.
The blue supergiant is a ferocious fusion reactor and it pushes a wind of plasma from its outer atmosphere into space.
Some of that material is pulled in by the companion to form a swirling disk of super-hot gas.
At a temperature of over a million degrees, the gas disk emits copious ultraviolet radiation and X-rays.
The companion’s gravity also distorts the outer envelope of the supergiant into a teardrop shape with its narrow end pointing towards the companion.
If we could follow the pointing teardrop and approach the swirling disk that marks the companion, we would see a small jot of utter darkness at its center: the black hole.
Einstein's Monsters is published by W.W. Norton and is priced at £18.99 in the UK.