What does the Moon have to do with our quest to understand the beginnings of our Universe?
Not a lot, you might think, considering the Moon is about 4.5 billion years old, whereas the Universe is thought to be 13.8 billion years old.
But the Moon has an important role to play in a branch of astrophysics that's hoping to unlock the secrets of exactly how the first stars formed, and how these stars evolved into the majestic galaxies we see today.
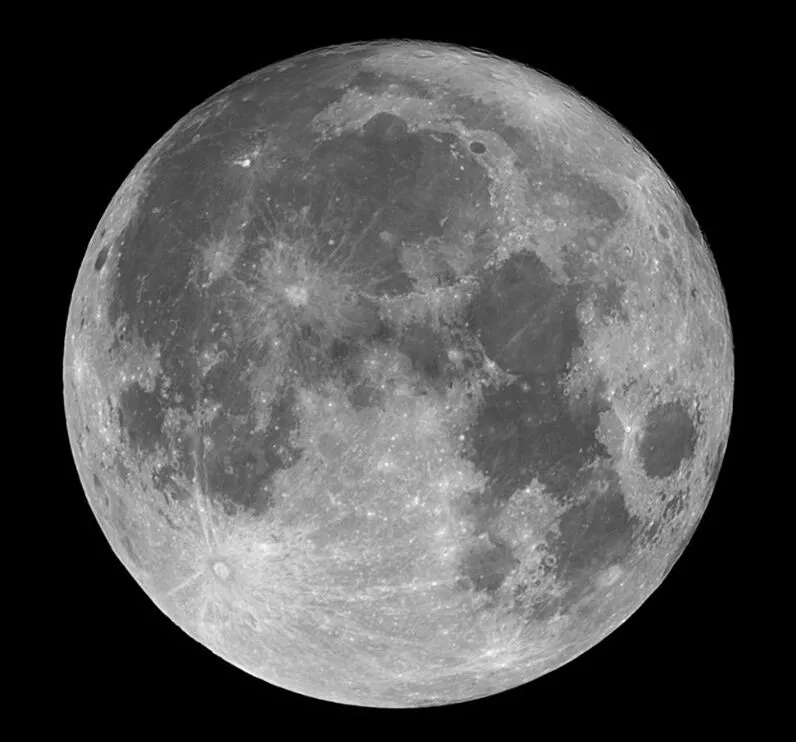
The Moon isn’t just Earth’s natural satellite. By blocking background galactic radio signals, it could be the key that unlocks the secrets of the early Universe.
Ben McKinley is a research fellow atCurtin University in Perth, Western Australia, specialising in understanding the complex structure and behaviour of radio galaxies.
We spoke to Ben to find out more about how our very own Moon can help in our quest to understand more about the evolution of the Universe as we see it today.
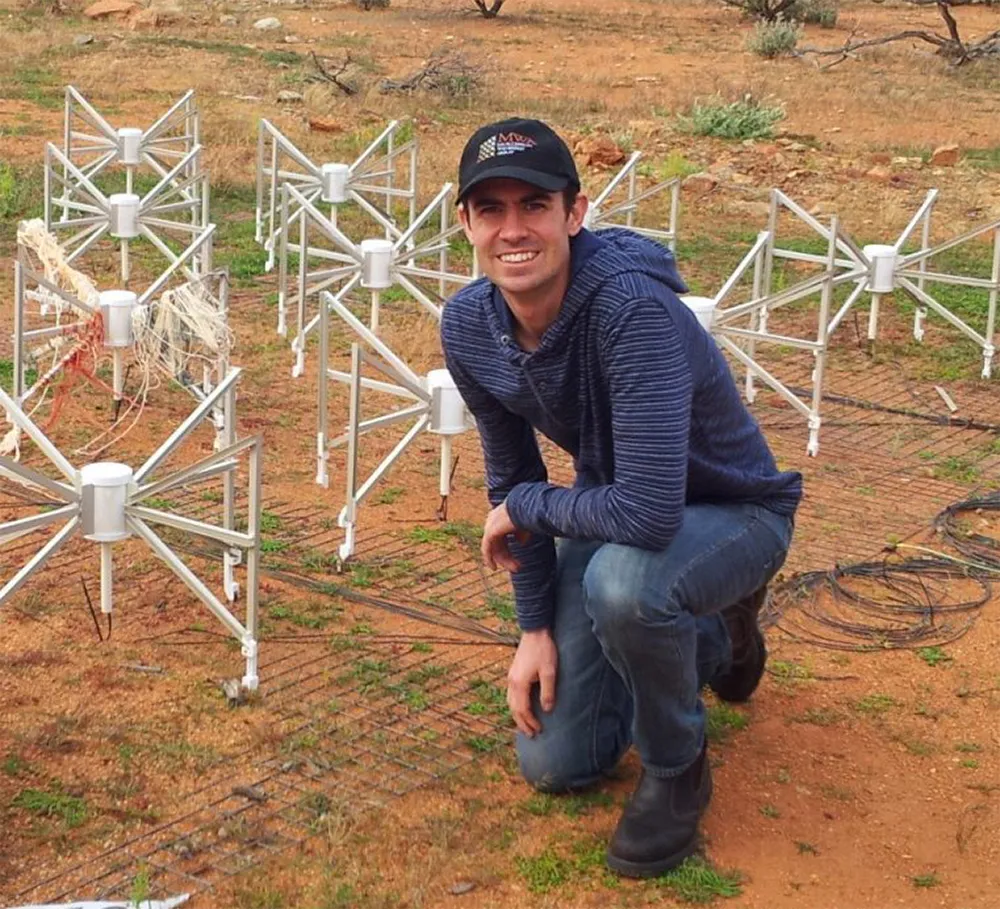
What does radio astronomy tell us about the early Universe?
When the early Universe expanded and cooled sufficiently, protons and electrons formed neutral hydrogen.
The first stars and galaxies then ‘reionised’ the hydrogen – that is, their photons interacted with the atoms, causing the electrons to separate. This is the Epoch of Reionisation.
Neutral hydrogen emits photons at a wavelength of 21cm.
But since the Epoch of Reionisation, the wavelength of these will have been redshifted (stretched by the expansion of the Universe) to between one and three metres, in the radio part of the electromagnetic spectrum.
How this redshifted 21cm signal looks tells us about these first stars and galaxies that reionised the hydrogen.
What are the challenges of detecting this signal from the Epoch of Reionisation?
This goal is fraught with difficulty, as the signal is weak and obscured by much brighter radio emissions in the foreground, coming from objects such as radio galaxies and electrons travelling at near light speed in our Milky Way.
This is where the Moon comes in; it occults the sky, providing variation to the otherwise featureless average radio signal we are looking for.
If we know the angular size of the Moon and its brightness as a function of frequency or wavelength, then we can use observations from the Murchison Widefield Array – a low-frequency radio telescope in Western Australia – to deduce the average background signal at different frequencies.
Why do you use the Moon?
The Moon acts as a known reference against which we can measure the average background of the sky.
The global average is otherwise invisible to the Murchison Widefield Array, which is sensitive only to angular variations of the signal.
In theory, any occulting shape could be used; the Moon just happens to be an ideal size in the sky and we think we know its brightness as a function of frequency.
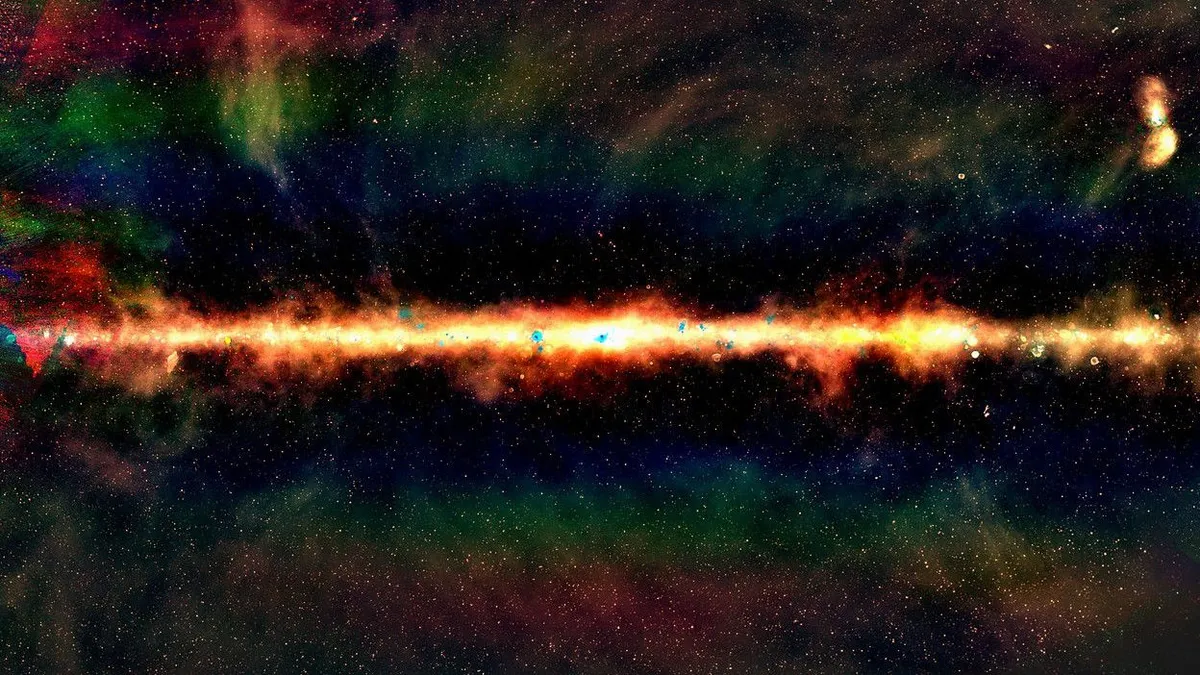
How does the Murchison Widefield Array help?
The Murchison Widefield Array could observe the Moon between 72MHz to 230MHz.
The telescope pointed at the Moon one night and on a subsequent night at the same local sidereal time, the same area was observed.
In the second observation the Moon would not be in the field of view as it would have moved away, due to its orbit around Earth.
This allowed us to subtract one set of images from the other, effectively removing the rest of the sky and leaving an imprint of the Moon.
The different images were then analysed to extract the global background sky that the Moon was occulting.

Did you have any problems?
The main problem was that we couldn’t assume the Moon was a black body [an object that absorbs radiation perfectly] of a constant temperature, because the Moon reflects radio signals originating from Earth back down to the telescope.
So what happens is you get a bright point in your images at the Moon’s centre, as it acts like a mirror.
This is especially a problem between the frequencies of 87MHz and 110MHz.
However, it turns out that reflections of sunlight and the heating of the surface by the Sun is a much smaller effect, and so for our purposes the phase of the Moon doesn’t matter.
How many observations have you made?
We’ve made observations over several nights to a total of around 250 hours and we are still in the process of analysing and publishing the data.
So far we only have one night each of an on–off Moon pair.
As we can’t cover the whole frequency range in one go we needed to split up the observations, such that we ended up with about 70 minutes ‘on-Moon’ time per frequency channel.
This was enough to demonstrate that our process worked and could measure the global foreground sky signal from the Milky Way – it was about 10,000 times brighter than the expected global 21cm signal.
This interview originally appeared in the November 2022 issue of BBC Sky at Night Magazine.