The first clues about aurorae beyond Earth came when the Pioneer and Voyager spacecraft passed close to the giant planets in the ’70s and ’80s. They found aurorae on Jupiter, Saturn, Uranus and Neptune.
Another spacecraft, the Pioneer Venus Orbiter, showed that ultraviolet emissions emanated from Venus’s night side, sometimes covering the entire hemisphere.
With the launch of the Hubble Space Telescope in 1990 came further discoveries.
Hubble revealed that Jupiter’s volcanic moon Io appeared to be ablaze when energetic particles struck its atmosphere.
Its sibling Ganymede was even more intriguing. Ganymede was known to have its own magnetic field, but in 1996 it was the first moon found to have an aurora – a ghostly green glow.
Hubble showed that the same mechanisms by which the aurora was created on Earth applied to Ganymede too.
Spanning the breadth of the electromagnetic spectrum, from X-rays through to radio emissions, the entire Solar System seemed to be aglow.
But did all these disparate phenomenon really count as aurorae?
Modern-day instruments are helping to answer that question.
We now know that aurorae appear, in one form or another, on each major planet of the Solar System (bar Mercury) as well as some of Jupiter’s moons.
The aurorae of Jupiter and Saturn are complex and powerful, while on Mars and Venus they’re very weak.
Unlike Earth, Venus and Mars have no molten, fluid core to generate a planet-wide magnetic field.On Mars, magnetic fields are instead found in small areas of surface crust.
“Mars has important, localised magnetic fields. Venus has no such crustal fields, and no aurora of that type has been observed there,” explains Dr François Leblanc of the French National Centre for Scientific Research.
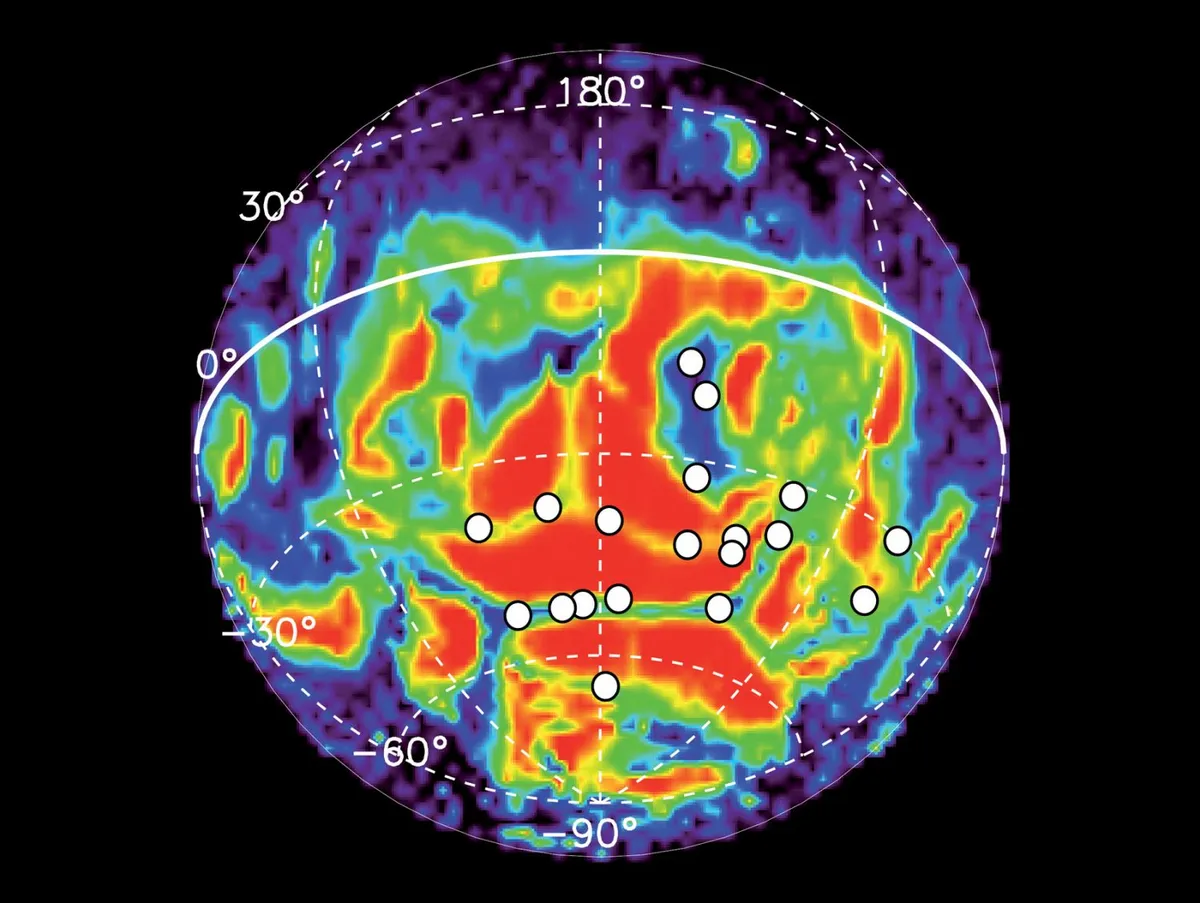
He says the presence of aurorae on Venus is controversial because the intensity of the emission is extremely weak.
Despite having no global magnetic field, the aurora on Mars is not disputed.
The European Mars Express probe was the first to discover Martian aurorae in 2005.It revealed that they’re very different to aurora on Earth.
Here, solar wind particles are trapped and funnelled by the magnetic field.
On Mars, they simply slam straight into the upper atmosphere.
They don’t occur at the poles but in patches above the surface corresponding to the crustal magnetic fields.
Mars Express observed in the ultraviolet range, but if you were to stand on the surface there is a chance you might see a visible glow, but it would be very faint.
That’s because the Martian atmosphere is 140 times thinner than Earth’s, so there’s less chance of charged particles striking atmospheric molecules.
“The aurora emissions on Mars are less bright and visible than on Earth,” says Leblanc.
Despite these differences the mechanism by which aurorae occur on Mars is similar to how they’re formed on Earth.
But mysteries still remain, says Leblanc. “One of the open questions today is how does this acceleration occur? We basically do not understand the range of energies.”
If Martian aurorae are weak, then Jovian ones are truly spectacular. So spectacular, in fact, that they’re several times larger than Earth itself.
Since 1998, Hubble has revealed that both Jupiter and Saturn’s powerful ultraviolet and infrared aurorae look like luminous ‘lassos’ of light.
Jupiter’s liquid, metallic hydrogen core acts like a dynamo.
It generates a magnetic field that’s 16 times more powerful than Earth’s, explains Dr Graziella Branduardi-Raymont of University College London’s Mullard Space Science Laboratory.
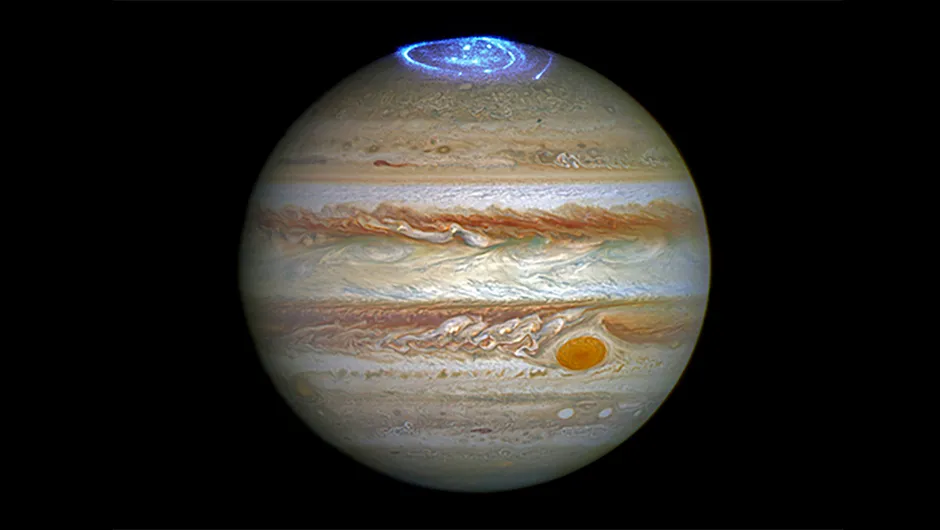
“Jupiter’s aurorae are made more powerful by its rotation,” she explains.
Solar wind particles penetrating the magnetosphere and accelerating toward the poles are what cause aurorae on Earth.
On Jupiter, most of these particles are in the magnetosphere already.
Some of the questions surrounding Jupiter’s aurorae may be solved thanks to the Sun.
Until around 2011, the Sun had been unusually quiet in terms of activity like sunspots and solar flares, and Jupiter’s aurorae were dimmer as a result.
This was an advantage for Branduardi-Raymont’s team, which looked at the planet using X-ray spectra obtained by the Earth-orbiting XMM-Newton and Chandra X-ray Observatories.
“The Sun wasn’t emitting as many X-rays – something that usually shows itself as scatter in Jupiter’s equatorial region and has nothing to do with aurorae,” she says.
The team saw something that could shed light on Jupiter’s auroral processes, although these results are yet to be published in a scientific journal.
In addition, there’s the question of whether there’s a correlation between aurorae on Earth and Jupiter.
In 2010 Branduardi-Raymont and her colleagues set about looking for this connection with the orbiting Chandra X-ray Observatory.
Since aurorae are caused by the compression of a magnetosphere by high-density solar wind, they monitored the Sun for coronal mass ejections.
This would generate a ‘wave’ of charged particles that would first pass Earth and then, two weeks later, arrive at Jupiter and start buffeting its magnetosphere – in the process increasing auroral activity.
Just as they are on Jupiter, particles are also present in Saturn’s magnetosphere.
The findings from NASA’s Cassini probe suggest that Saturn’s aurorae occur in a similar way to those on Earth, and that they brighten depending on which part is facing towards the Sun.
A discovery made using the Hubble Space Telescope showed that the auroral process is connected to other activity on the planet.
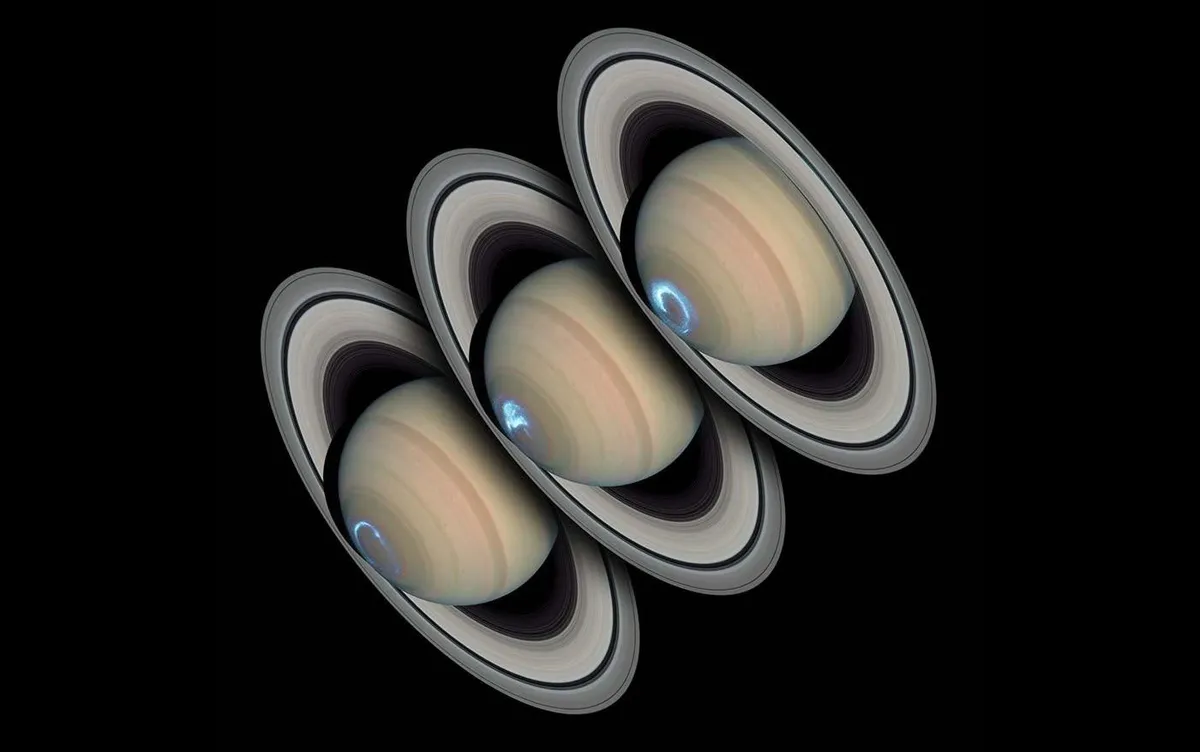
It’s long been known that Saturn emits radio waves from its polar regions every 11 hours – the length of Saturn’s day.
The mystery was that the timing of these pulses had changed over the years.
It was highly unlikely that the planet’s speed of rotation had changed, so what was causing them?
In August 2010, Dr Jonathan Nichols of the University of Leicester linked the pulses with an ultraviolet glow illuminating the upper atmosphere near the poles.
The same process driving the aurora could also be creating the radio waves.
In the meantime, the study of planetary aurorae continues.
Uranus and Neptune are known to have well-defined aurorae when viewed in ultraviolet.
To find out more, astronomers are looking forward to the launch of the James Webb Space Telescope.
With a primary mirror area over 5.5 times larger than Hubble’s, it will examine a greater range of wavelengths, from near infrared to deep infrared, and potentially open up a new area of auroral discovery as far as Uranus and Neptune are concerned.
It will even make Jupiter’s atmosphere darken in images, leaving just the aurorae.
What is an aurora?
On Earth, visible aurorae occur when charged particles from the Sun – in the form of the solar wind and coronal mass ejections – buffet the planet’s magnetic field.
It appears that for a true auroral emission, these charged particles must be accelerated in the magnetic field to collide with atmospheric molecules.
On Earth this happens in the ionosphere, some 80km above the ground.
The molecules ionize by losing an electron. When they regain an electron (‘recombination’), they emit a photon of light.
Recombination of oxygen gives green and reddish light, while nitrogen gives blue or red.
The process is harnessed for artificial lighting by sodium and mercury vapour street lamps.
This article originally appeared in the January 2011 issue of BBC Sky at Night Magazine.
Read more: