The Kuiper Belt is a ring of rocky, icy bodies that begins 30 AU from the Sun at the orbit of Neptune, and extends outwards in the plane of the Solar System for several thousand AU at least. It’s estimated there are roughly 100,000 objects larger than 100km in diameter in the Kuiper Belt, but only the largest and closest objects can be directly detected with present-day surveys.
For every asteroid in the main belt between Mars and Jupiter, there are roughly 1,000 Kuiper Belt Objects (KBOs) of similar size, spread over a volume of space at least 1,000 times that of the Asteroid Belt.
There are likely to be a few more objects the size of Pluto and there’s room for something much larger, but not in the inner regions of the belt where a large body would be easy to detect.
A few other large Kuiper Belt objects have been found such as Eris, Makemake, Haumea and Sedna.
And yet, the mass of all these objects added together is still only one-tenth of the Earth’s mass. It’s likely that many more bodies were present when the Solar System formed 4.5 billion years ago.
For more info on calculating these mind-blowing numbers, read our guide on how astronomers measure distance in space.
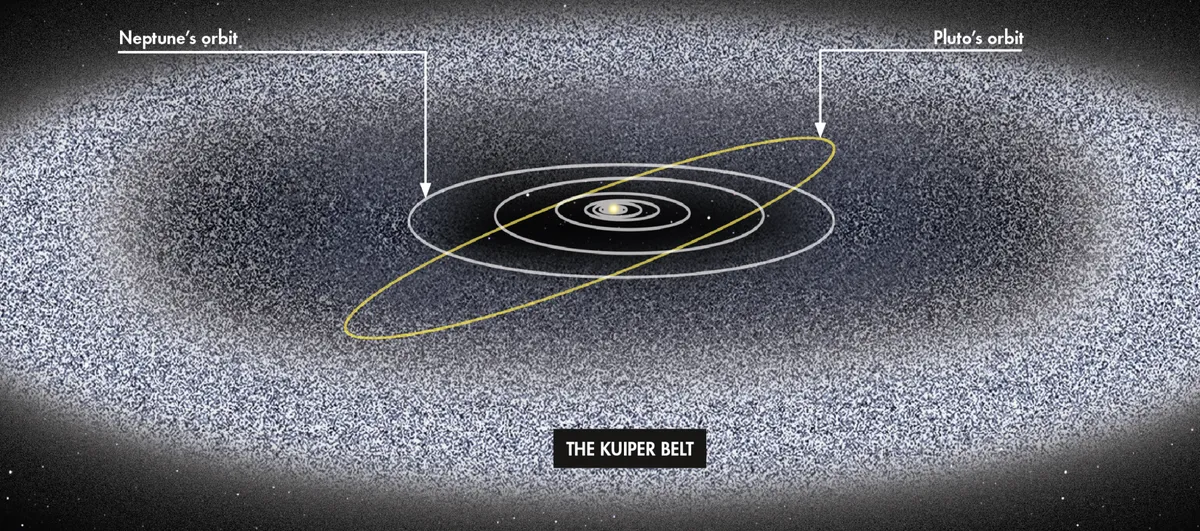
How was the Kuiper Belt discovered?
A number of scientists such as Fred Leonard in 1930, Kenneth Edgeworth in 1943, Gerard Kuiper in 1951 and Fred Whipple in 1964, speculated that Pluto might not be alone in the outer Solar System.
Kuiper went a step too far, though, and actually predicted that the region where we discovered the Kuiper Belt formed full, but was then emptied by destabilising perturbations by Pluto.
These assertions, much like those of Nostradamus, had little impact when they were made because they are too vague to be observationally tested.
It was not until 1980 that Uruguayan astronomer Julio Fernández argued more convincingly that short-period comets might come from a disc-shaped region beyond Pluto, instead of from the more distant Oort Cloud as previously proposed.
Even this made little stir, perhaps because of the dubious history of empty predictions made by Percival Lowell and others after him, such as Lowell’s supposed observations of canals on the surface of Mars.
The simple truth is that out of sight is out of mind, even for astronomers. Why think much about something that probably isn’t even there?
In the end, the Kuiper Belt was discovered not in response to any meaningful prediction but because, like Tombaugh, we were looking.
The trigger for the discovery of the Kuiper Belt was a simple question: why is the outer Solar System so empty?
In the mid 1980s we knew that the inner Solar System was full of planets and asteroids, but the outer Solar System held only Uranus, Neptune and Pluto.
I guessed that the answer might simply be that nobody had carefully looked.
Graduate student Jane Luu and I began our search in 1986, but rather than searching for a Kuiper Belt beyond Neptune, we were looking for any object beyond Saturn.
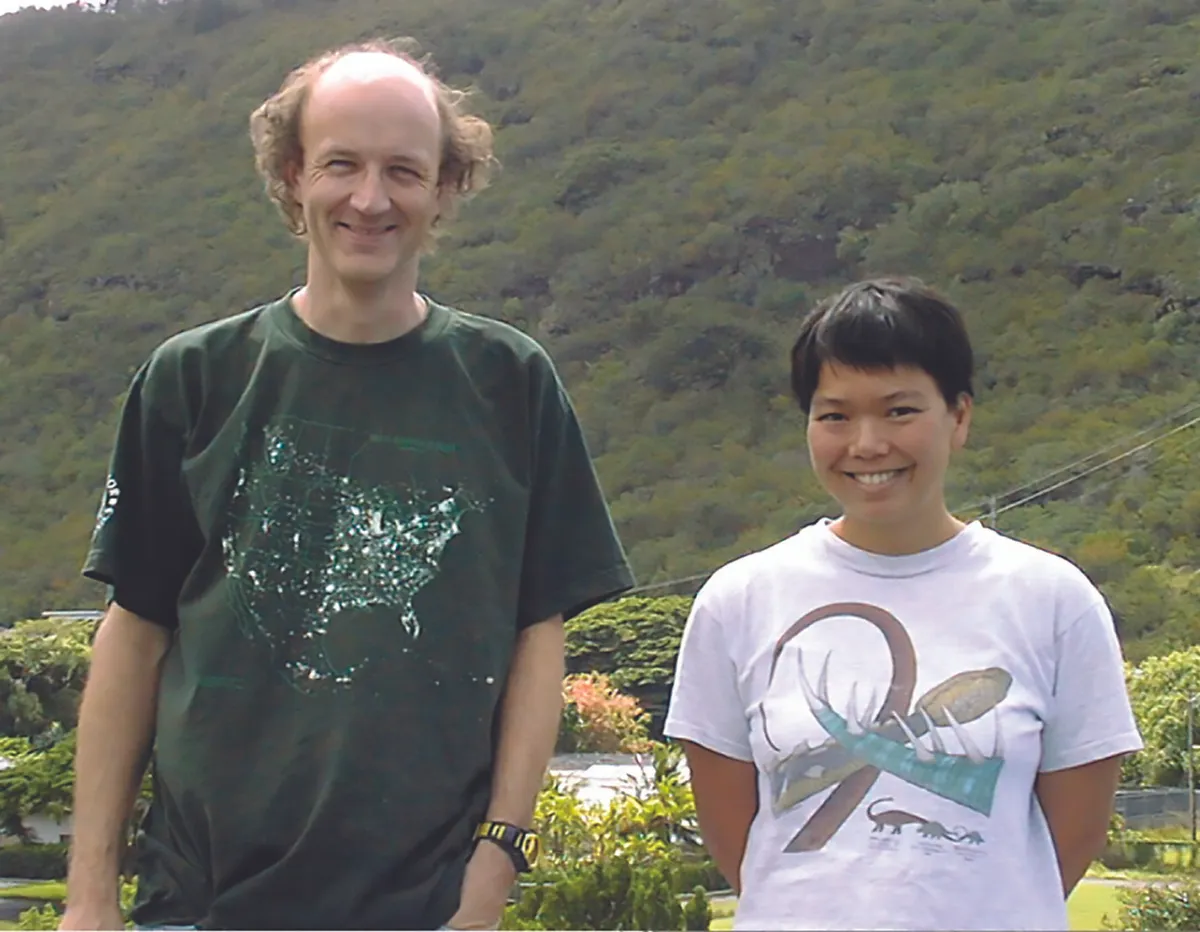
We didn’t know how difficult it would be or how long it would take. Six years later, sitting in the cold, thin air atop Mauna Kea in Hawaii using the 2.2m telescope, we noticed something.
We were taking four consecutive images of a given patch on the sky. By carefully aligning the images and then flipping between them on a computer, we could distinguish moving objects from the thousands of stars and galaxies in each picture.
We discovered uncountable numbers of asteroids this way. But we were interested in finding objects beyond Saturn, and we expected that these would creep much more slowly westward and would be faint.
On 30 August 1992, at about midnight, we saw something that had exactly the characteristics we wanted.
I noticed it by comparing the first two of four 20-minute exposures. 20 minutes later, the 3rd image confirmed the direction and slow motion and, 20 minutes after that, the 4th image left us briefly dazed with excitement.
Quickly, we calculated the distance from the speed, then the size from the distance and the brightness.
By the end of the night we knew that 1992 QB1 was about 250km in diameter and 50 AU from the Sun, by far the most distant Solar System body ever seen, and we realised that thousands of similar objects were waiting to be found.
A few hours later and a mile below the summit, we celebrated with a big, greasy breakfast.
We did not succeed until August 1992, when we found 1992 QB1, the 2nd Kuiper Belt object (KBO) ever found.
6 months later we found another object and then, over the next few years, the floodgates opened.

After the flood
So, what have we learned since? Firstly, it is now clear that Pluto is a big KBO.Its peculiarly inclined, elliptical orbit suddenly makes sense – it is just like the orbits of innumerable other KBOs.
Secondly, the Kuiper Belt is an enormous, deep-freeze repository holding the most primitive material in the Solar System.
With temperatures only a few tens of degrees above absolute zero, even very volatile ices like carbon monoxide that cannot survive near the Sun are frozen solid in the Kuiper Belt.
Icy objects leaving the belt are batted around the Solar System by the giant planets, leading to some being ejected to the interstellar medium never to be seen again, while others are captured by Jupiter.
Ices in KBOs deflected near the Sun vaporise to create comets, with their familiar tails and coma.
Thirdly, we found that while the KBOs are very numerous, their combined mass is only a modest 0.1 Earth masses.
This is so small that it would be difficult for the observed objects to have accreted, even over the age of the Solar System.
The solution seems to be that the Kuiper Belt started out being much more massive than it is now, perhaps containing 20 or 30 Earth masses instead of 0.1, but then lost almost all of it. Where did it go?
The answer may lie in two other observational discoveries from the 1990s. We found that the Kuiper Belt is a thick disc, more like a doughnut than a sheet of paper, showing that the belt has been unexpectedly ‘puffed up’ since it formed.
And we found, much to everybody’s surprise, that the orbits of KBOs are divided into several distinct groups.
In one of these called the ‘resonant KBOs’, the orbital periods are simple variations of the 164.8 year orbital period of Neptune.
For example Pluto’s period (247.9 years) corresponds to two orbits for every three of Neptune’s.
Neptune and Pluto are said to be in the ‘3:2 resonance’, along with thousands of other objects.
Many other resonances (e.g. 2:1, 4:3, 1:1) are also occupied. But what made the Kuiper Belt so puffy, and why are there so many resonant KBOs?

All linked together
University of Arizona dynamicist Renu Malhotra gave us the answers. Resonant orbits prevent close, destabilising encounters with Neptune, allowing resonant KBOs to persist because they never tangle with ‘the big guy’.
Malhotra found resonant KBOs were trapped because Neptune’s orbit slowly expanded, from near 15 or 20 AU in the beginning to 30 AU now.
As the planet scooted outwards, it trapped some of the planetesimals beyond it into resonant orbits.
But the planets pull on each other by gravity so, if Neptune’s orbit changed, they all changed. This ‘radial migration’ of the planets has revolutionised our thinking about the Solar System.
In place of the old and rather boring clockwork Solar System in which the planetsheld their orbits and moved predictably for billions of years, we now recognise a much more chaotic and harder to follow history.
For example, simulations show that if radial migration caused two of the major planets to fall into a resonance of their own, this would have caused the entire architecture of the Solar System to be catastrophically upset.
If this happened in the past then the initially massive Kuiper Belt would have been disrupted, showering the Solar System with debris and causing a swarm of giant impacts, perhaps recorded in the large basins that make up the ‘face’ on the Moon.
All that would be left would be the puffed-up Kuiper Belt remnant that we see today.
As a result of all this, the significance of the New Horizons encounter with Pluto has changed since the mission was first imagined in the late 1980s.
Instead of visiting the last, most peculiar planet, we found that we had visited a large but otherwise unremarkable Kuiper Belt object.
Even before New Horizons we knew a lot about Pluto, including its mass, diameter and density, the composition of its surface ices, the existence, nature and variability of its atmosphere and the properties of its satellite system.
But the New Horizons encounter has taken it to the next level by transforming Pluto from an astronomical object to a geological one, rich with surface detail that can never be detected from Earth.
David Jewitt is a professor at the University of California Los Angeles. He became interested in astronomy after watching a spectacular meteor shower in his native Enfield.
This article originally appeared in the November 2015 issue of BBC Sky at Night Magazine.