The most sensitive instrument in the history of astronomy is going to be built in deep underground caverns and tunnels, a few hundred metres beneath Earth’s surface. It may sound crazy, but it’s true.
The Einstein Telescope (ET) isn’t going to collect light photons and won’t provide us with stunning images of nebulae and galaxies.
Instead, the almost €2bn–facility will register gravitational waves, the infinitesimal ripples in spacetime that propagate through the Universe at the speed of light as a result of the collisions and mergers of ultra-compact neutron stars and gluttonous black holes.
The Einstein Telescope will form a giant equilateral triangle, measuring 10km on each side.

Powerful beams of laser light will travel up and down these arms, bouncing off mirrors to gauge the length of the arms to see if they change by even a fraction the width of an atomic nucleus.
When such a change does occur, it could mean a gravitational wave has passed through, briefly warping the space around the detector.
"Our target is to detect colliding black holes throughout the whole observable Universe," says Michele Punturo, co-chair of the international ET steering committee.
What are gravitational waves?
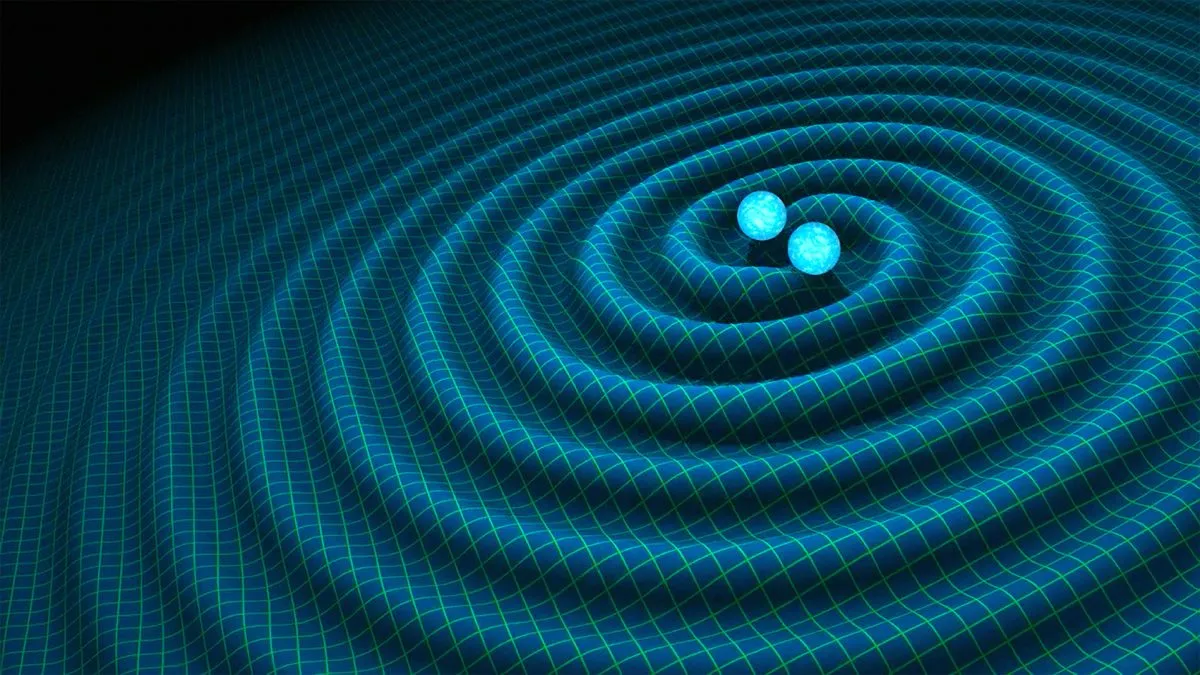
Gravitational waves were first described over a century ago by Albert Einstein.
His general theory of relativity implied that spacetime could bend, warp, expand or contract, like a four-dimensional rubber sheet.
Einstein also predicted that accelerating masses create tiny undulations in the very fabric of spacetime, alternately stretching and compressing everything on their paths.
However, even the largest masses and the strongest accelerations would produce gravitational waves with amplitudes much smaller than an atomic nucleus. Einstein was convinced they would never be detected.
60 years after his death, in 2015, Einstein was proven right on the existence of gravitational waves, but wrong on their non-detectability.
Indeed, the two highly sensitive detectors of the Laser Interferometer Gravitational-Wave Observatory (LIGO) registered the spacetime ripples of two colliding black holes at a distance of 1.3 billion lightyears.
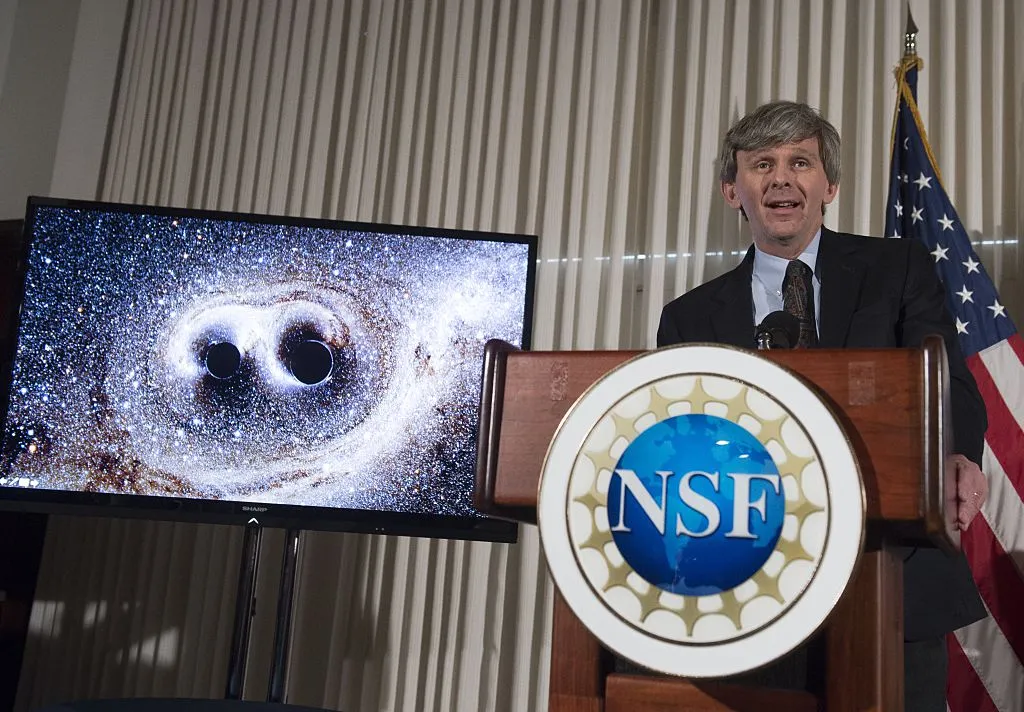
Since that first discovery of gravitational waves, both the US-based LIGO and the European Virgo detector in Italy have observed dozens of events, including tell-tale signals from colliding neutron stars, smash-ups of black holes and neutron stars, and black holes with unexpected masses – maybe a result of earlier mergers.
"Being able to learn more about these fascinating objects is just incredible," says theoretical astrophysicist Samaya Nissanke of the University of Amsterdam.
"The observations have had an enormous impact on a wide variety of scientific fields, including astronomy, physics, cosmology and general relativity.
"And what strikes me most is how fast we’ve grown completely used to actually observing black hole mergers. It’s amazing."
So far, only one gravitational-wave event (known as GW170817, due to the merger of a black hole and a neutron star) has also been observed by regular telescopes, but Nissanke, who is an expert in this new field of multi-messenger astronomy – meaning she studies the Universe using methods other than just electromagnetic radiation – isn’t concerned about the lack of more detections with electromagnetic counterparts.

"We were lucky in the case of GW170817," she says. "It’s not easy, but soon enough, we’ll be getting more sensitive detectors, better ‘sky localisations’, and more powerful sky surveys. It will happen again."
Nissanke looks forward to the Einstein Telescope, which will be much more sensitive than either LIGO or Virgo.
Reaching out to distances 10 times as large, the instrument will yield a thousand-fold increase in the number of gravitational-wave events, as volume goes up in proportion to distance cubed.
"It’s phenomenal," she says. "The Einstein Telescope will be able to detect many thousands of events per year."
Building the Einstein Telescope underground

This will only be possible through a whole set of different improvements, explains Sheila Rowan, director of the Institute for Gravitational Research at the University of Glasgow.
"The instrument will be built underground to diminish seismic noise," she says.
"Some of the core components of the detector will be cooled to 10˚C above absolute zero. Because of these low temperatures, the mirrors need to be made out of ultra-pure crystalline silicon instead of more regular glass or quartz.
"We’re also reaching out to lower-frequency waves," which increases the instrument’s ability to detect in-spiralling binary black holes at an earlier stage.
Last but not least, each of the three arms of the Einstein Telescope will be 10km long, greatly increasing the sensitivity compared to LIGO’s arm length of just 4km.
Each LIGO and Virgo detector is fitted with just one interferometer, located where the two arms of the L-shaped detector meet. But the triangle-shaped Einstein Telescope can hold an interferometer at each of the three vertices.
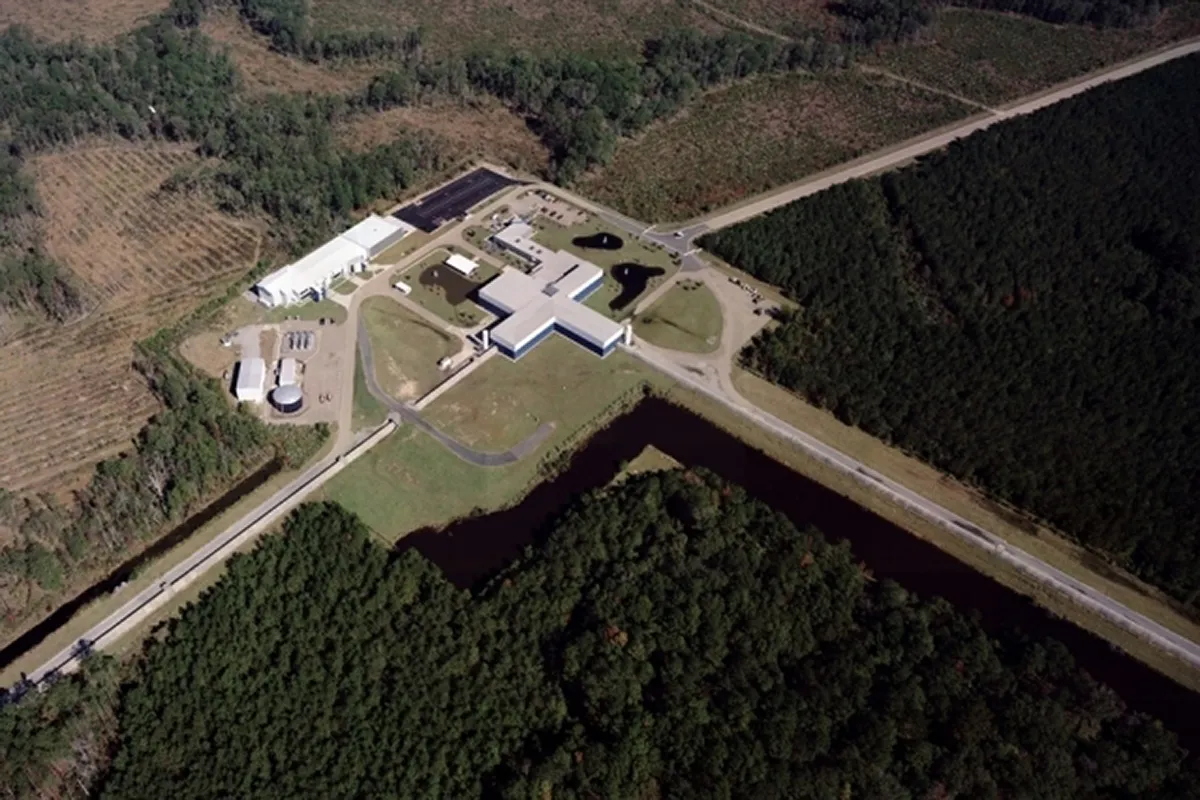
In fact, it will hold two interferometers at each vertex, looking for gravitational waves of lower and higher frequencies, as the lower the frequency the larger the mass of the source.
The Einstein Telescope’s multi-interferometer setup will also make it possible to study the polarisation of the spacetime ripples, providing useful information about how the black holes were spinning as they collided.
Punturo notes that the current design of the Einstein Telescope is still rather preliminary.
It could change in the future, perhaps in response to the plans for an American counterpart, known as the Cosmic Explorer, which is still at an earlier stage of development.
"No, we don’t call it a competitor," he says. "We are partners in science, and the best science will be achieved by mutual cooperation between various facilities."
The same is already very much true for LIGO and Virgo (and other gravitational-wave detectors).
The ET Pathfinder
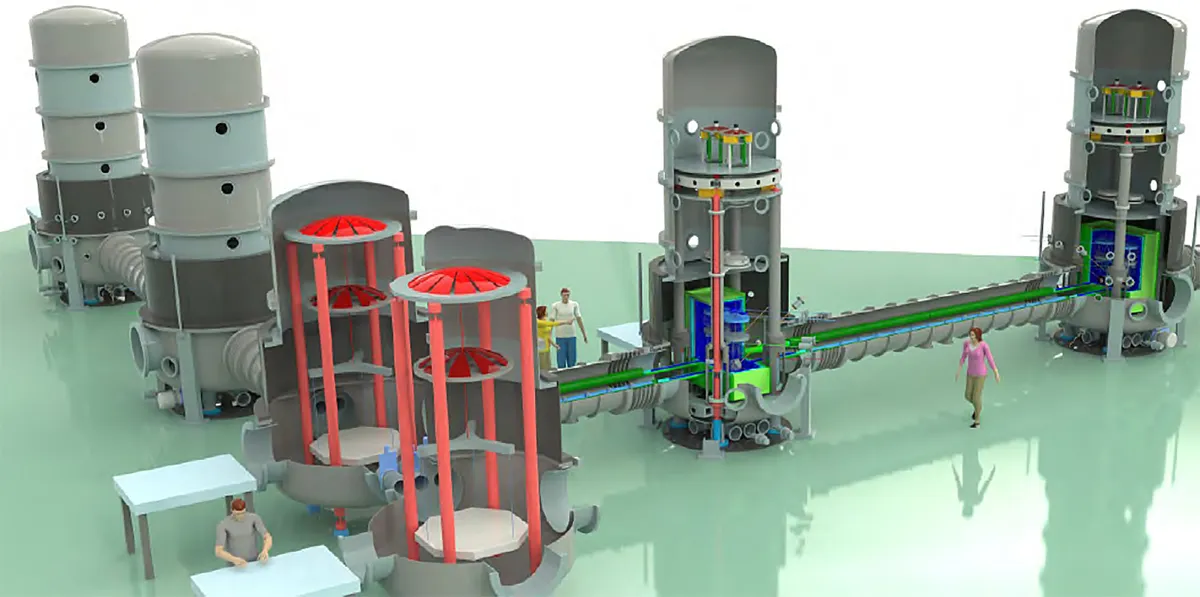
There’s no doubt, however, that many new technologies will have to be incorporated in the Einstein Telescope.
That’s why scientists are constructing the €15m ET Pathfinder – a testbed for cryogenic components, silicon mirrors, novel suspension mechanisms and new types of lasers.
The scale the detectors will be working on are so small that quantum mechanics will start having an effect, so they also need to trial smart measurement techniques that will diminish the effect of the Heisenberg uncertainty principle (which concerns the difficultly of measuring the position and momentum of a particle at the same time).
"We expect ET Pathfinder to be up and running in late 2022," says Stefan Hild of Maastricht University in the Netherlands, the leader of the construction effort.
ET Pathfinder is being built in a former newspaper printing plant that has been converted into a high-quality 1,000-square-metre clean room with a 50cm-thick floor supported by 170 concrete piles measuring 6m-long and 30cm in diameter.
With arm lengths of just 10m or so, ET Pathfinder won’t be able to actually detect gravitational waves, but most components of the testbed facility are fully functional.
"Over the next months, we will be building quite a lot of things," says Hild, who received a European Research Council Advanced Grant of over €2m for developing new detection methods.
Where will the Einstein Telescope be built?
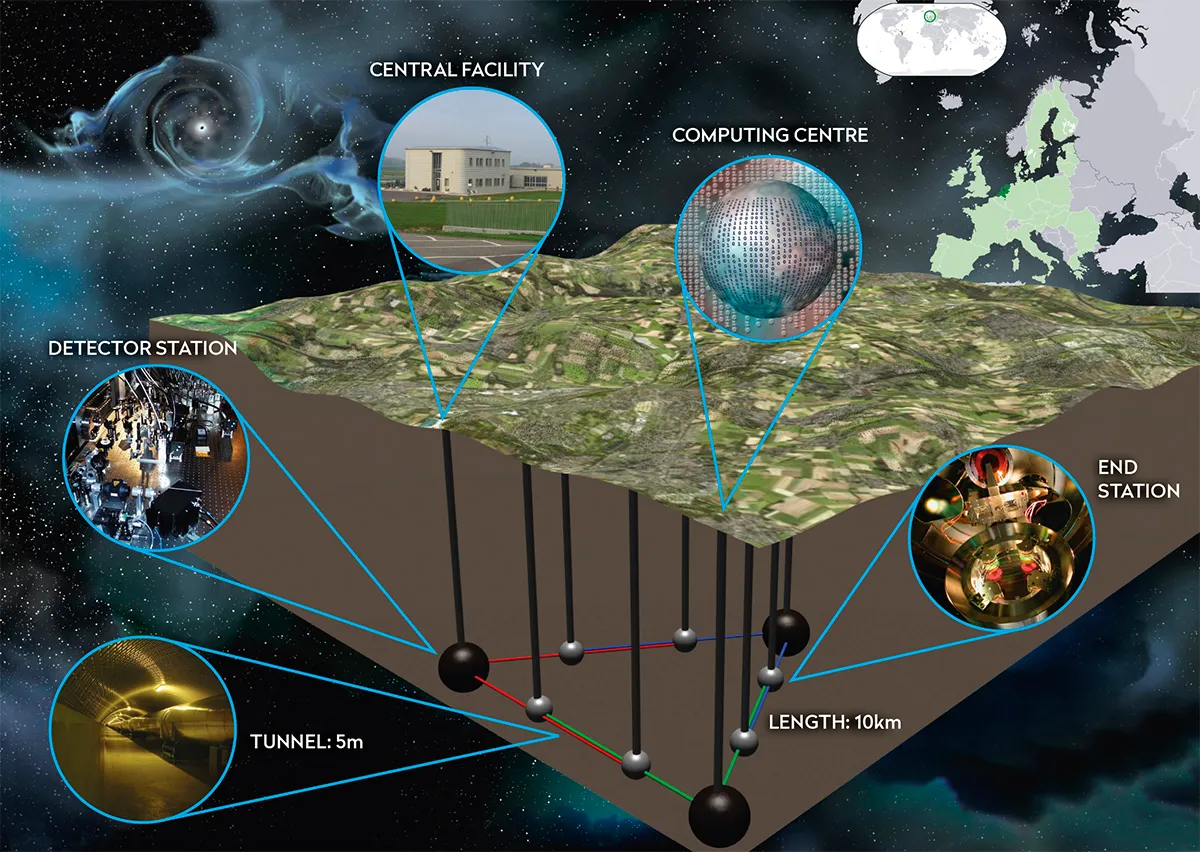
Maastricht, in the Netherlands, is also close to one of the two candidate locations for the full-scale Einstein Telescope: the underground facility could possibly be built beneath the tripoint of the Netherlands, Belgium and Germany.
The other candidate site is located in the northeast of the Italian island of Sardinia.A final decision is not expected before 2024 or 2025.
"The term 'best place' is a very complex one," explains Punturo. "It involves not only science, technology, and geology, but also politics and logistics."
Both sites are known for their low level of seismicity (even minute tremors could disturb the measurements), but Sardinia has a low population density. "Humans are noisy," says Punturo.
In the summer of 2021, the European Strategy Forum on Research Infrastructures (ESFRI) included the Einstein Telescope in the plans of its 2021 Roadmap, underscoring the importance of the new facility for European science.
After the site has been selected and the final design has been approved, construction of the Einstein Telescope would commence in 2026.
According to Punturo, the first preliminary measurements could be made in the early 2030s, while the start of full operations is anticipated in 2035.
"It will be a precision machine for black hole and neutron star mergers that are relatively nearby," says Punturo, "and a discovery machine for the distant and early Universe.
"We may even detect gravitational waves from primordial black hole collisions that happened during the cosmic Dark Ages, before the formation of the very first stars and galaxies."
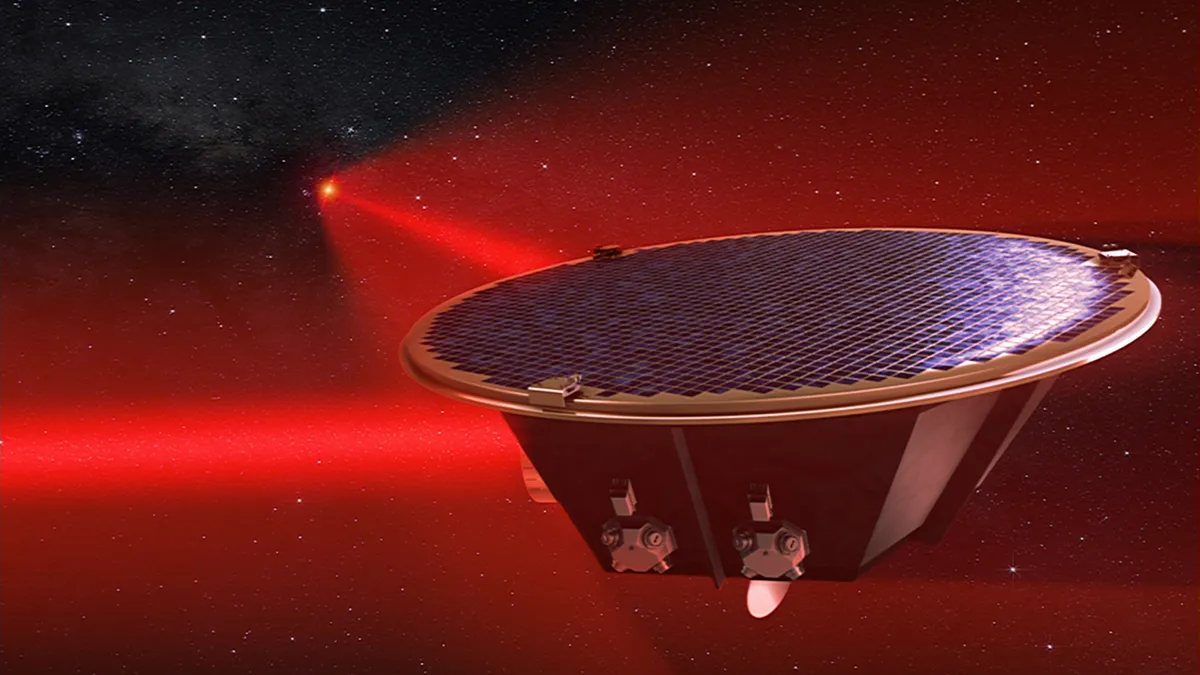
In 2034, the European Space Agency also plans to launch its Laser Interferometer Space Antenna (LISA), a space-based gravitational wave detector.
LISA operates at a much lower frequency, enabling the detection of merging supermassive black holes in the cores of distant galaxies.
Indeed, the two facilities will be very complementary in opening up a new window on the Universe. "Together, they will hopefully find a lot of missing parts of the giant cosmic jigsaw puzzle," says Nissanke.
"It’s just mind-blowing to imagine how astronomy will look 20 years from now."
This article originally appeared in the September 2021 issue of BBC Sky at Night Magazine.