When large stars reach the end of their lives they explode in a supernova, one of the most sudden and violent events in the sky.
Large stars live fast and die young. They, like stars of all sizes, create light by converting hydrogen to helium in a process known as nuclear fusion.
But in the most massive stars this process is rapidly accelerated, meaning that they can burn through their stores of hydrogen gas in as little as a few million years.
Compare this to the Sun, which has a lifespan estimated to be 10 billion years.
How a supernova happens, step-by-step
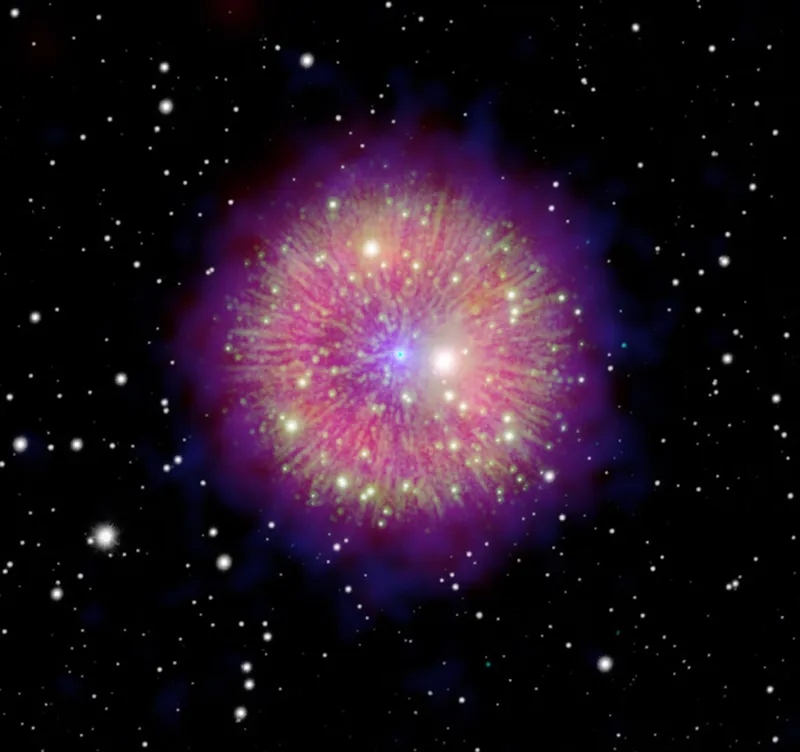
Stars live in a delicate balance between two opposing forces: gravity trying to make them collapse inwards and thermal pressure of nuclear fusion exerting outward pressure.
As the hydrogen in the core gets used up, the star begins to convert helium into heavier elements.
At first lithium and oxygen, before working all the way up the periodic table to iron.
Heavier elements cause the core to become more dense, increasing its gravitational pull.
Fusion reactions also release less energy to balance this out.
All the star has to act against gravity is electron degeneracy pressure, the resistance to having more than one electron in the same place at the same time.
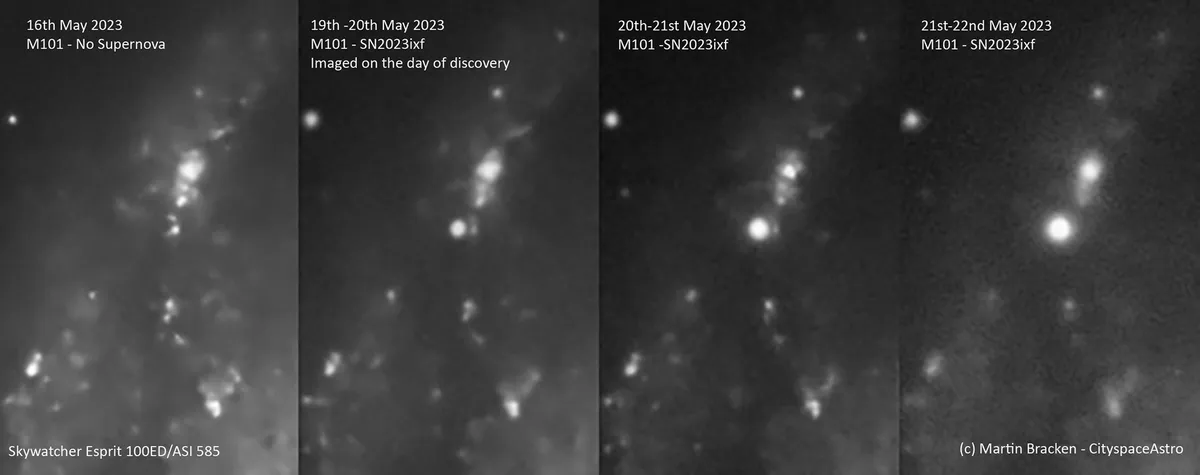
When the core reaches a critical density of 1.4 solar masses, known as the Chandrasekhar limit, even the plucky electrons can’t keep up the fight.
The star’s core collapses in a matter of seconds, quickly followed by the outer layers of gas rushing in at as fast as 25% the speed of light.
The core continues to collapse until the resistance between atomic particles stops it from collapsing any further.
All the atomic nuclei are tightly packed together by now, creating a solid surface.
Normally, this tightly packed ball remains as a neutron star.
If the core is massive enough it may continue to collapse even further, creating a black hole.
The gas that was rushing in at incredible speeds strikes against the now solid surface of the neutron star.
It rebounds in a massive shockwave that ends in a huge explosion – the supernova.

How astronomers observe supernovae
A supernova creates a huge amount of light for a short amount of time.
Astronomers hoping to study such fleeting events look for points of light that suddenly appear in the night sky.
Originally this was done by eye, and some amateur astronomers still search for supernovae at the eyepiece.
But most professional supernova surveys nowadays use automated systems to image the sky, searching for ‘stars’ that weren’t there the night before.
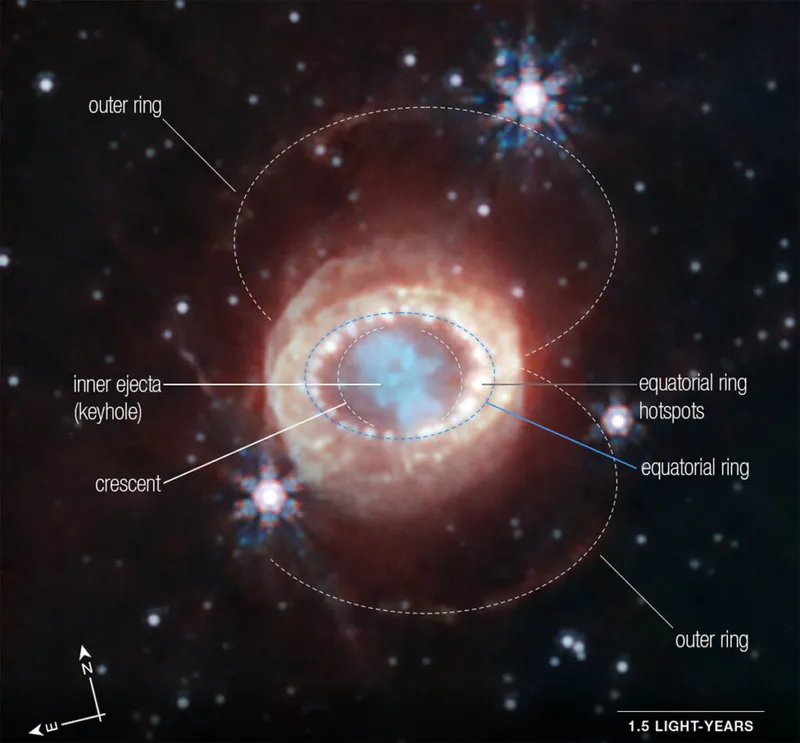
Not everything they find is an exploding star, however.
Some explosions are dimmer nova, where the interaction between a pair of stars causes one to temporarily flare up.
In August 2017, researchers observed a kilonova for the first time, a much brighter explosion caused by the collision between two neutron stars.
These are thought to be the origin of all naturally occurring elements heavier than iron.
More common is for astronomers to observe a supernova remnant, which is the expanding glowing cloud of cosmic gas and dust left over from the explosion.

How astronomers observe a supernova
To uncover what kind of nova an explosion might be, researchers have to watch how the light from the bright, new object changes.
But they need to be quick, because after the explosion the light quickly fades from view.
Once a new supernova is found, astronomers notify all the telescopes in the world that might be able to observe the star.
They take brightness measurements across different wavelengths and use spectroscopy to discern which elements were in the star when it exploded.

These elements don’t just disperse into the Universe, but instead form a type of nebula called a supernova remnant.
These nebulae are rich in hydrogen gas, which clumps together to form the next generation of stars.
Meanwhile, the heavy elements coalesce together, eventually forming planetary systems around the stars in the nebula.
By studying supernovae, researchers not only grow to understand the life cycles of massive stars, but also the origins of planets too.
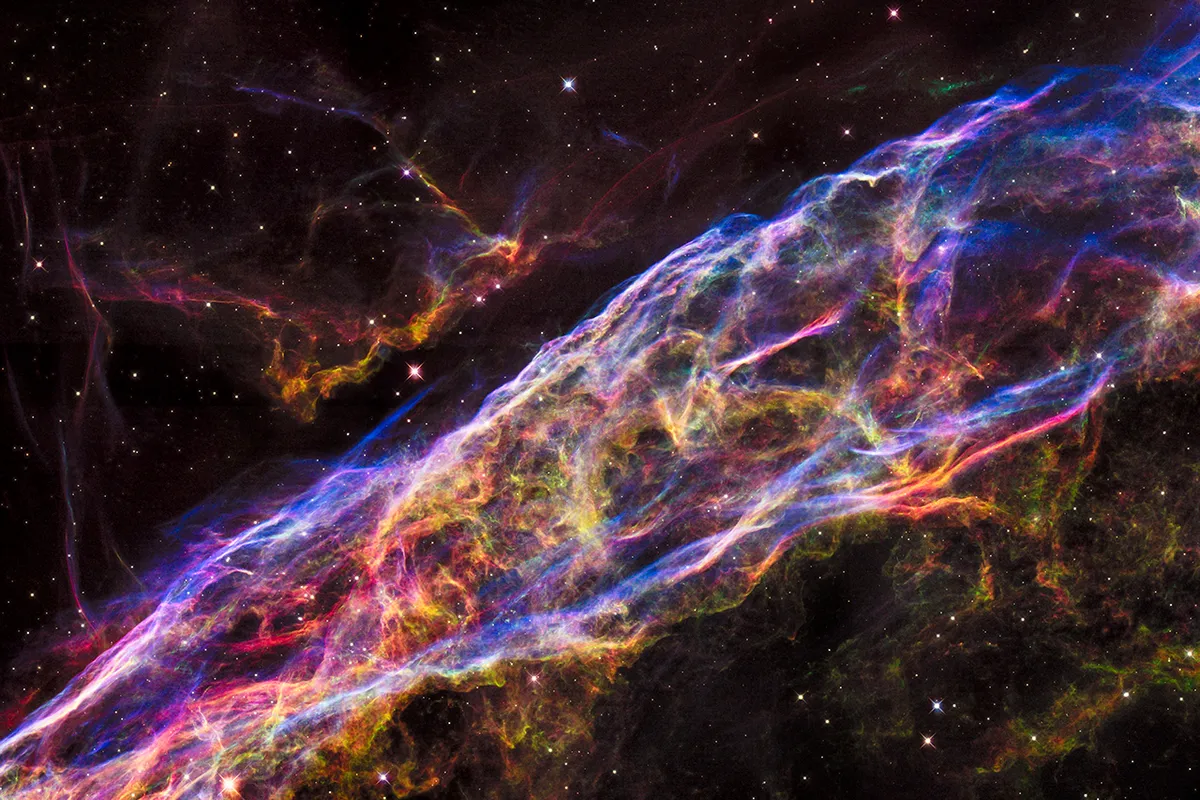
Types of supernova
The state of a star before it collapses can change the supernova it creates.
There are two main categories of supernova, depending on how much hydrogen is seen in the afterglow.
Type I supernovae contain a small amount of hydrogen, Type II contain more.
Type II supernovae originate from the biggest stars, which have a very short lifespan so their outer layers of hydrogen gas are still intact when they explode.
Slightly smaller stars might lose this layer over time, either through their own solar wind or because a neighbouring star strips away the gas.
In Type Ib supernovae, the outer layer of hydrogen has been lost, while Type Ic have shed their helium as well.
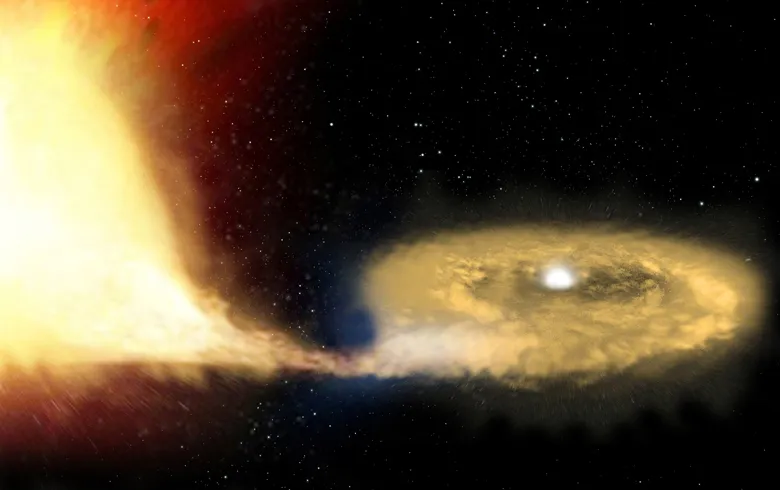
Type Ia supernovae are created by smaller stars that get locked in a tight binary pair with a smaller white dwarf.
The white dwarf steals material from its neighbour, until it reaches the critical mass to cause an explosion.
As an explosion’s brightness is linked to the star's mass, Type Ia supernovae always have the same luminosity.
So astronomers can observe how bright it appears, compare to how bright it really is, and use that information to measure its distance.