The Sun is centre of our Solar System, a scorching sphere of hydrogen and helium that plays gravitational host to Earth and all the other planets, dwarf planets, moons and space rocks.
Solar scientists estimate our Sun is about 4.5 billion years old. After the Sun's formation, a disc of cosmic debris was left encircling the young star.
Out of this material, the planets of the Solar System were born.
- View images of the Sun, or find out how to safely observe the Sun.

Earth lies about 150 million km from the Sun, and this distance - known as an Astronomical Unit - is used to measure large-scale distances between other bodies.
Neptune, for example, is about 30 AU from Earth.
The Sun has a radius of about 696,000km and is big enough to hold over 1 million Earths!
Without the Sun, we would be nothing: it is the source of life on our home planet.
And yet, for those of us who aren't astronomers or solar scientists, it can sometimes feel like we know more about Mars or the gas giants Jupiter and Saturn than our own host star.
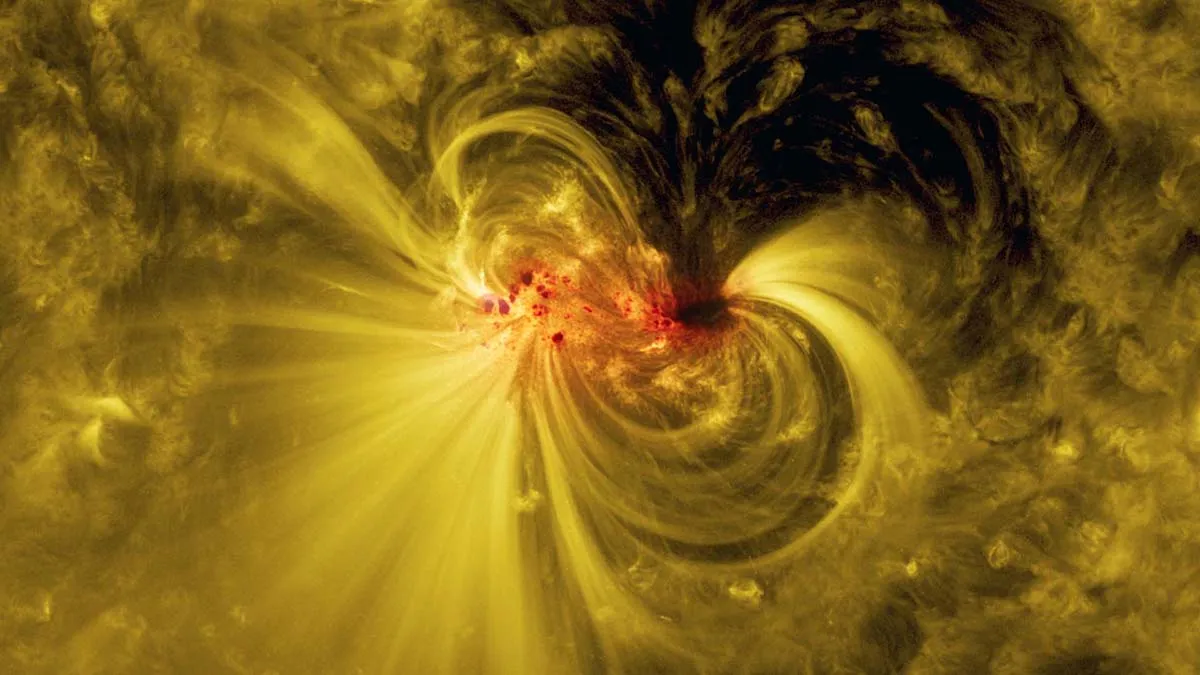
There is much yet to be discovered about the Sun, but solar astronomers have been able to answer some fundamental questions about its structure, what makes it tick and its influence on the rest of the Solar System.
Here are some of the biggest questions about the Sun and solar science, and their answers.
1
What sort of star is the Sun?

The Sun is classified as a G-type main sequence star. The ‘main sequence’ part of the name refers to the fact that the Sun is at a stage in its life when the primary fuel source is the conversion of hydrogen to helium.
Stars in this phase lie on what we call the main sequence of the Hertzsprung-Russell (HR) diagram (seen above).
This diagram is a graph of stars’ absolute magnitudes versus their surface temperatures.
Stars spend the majority of their lives on the main sequence; the exact duration depends on the mass of the star.
And the main sequence is the most prominent curve on this diagram, forming a slanted ‘S’.
The main sequence is not a sharply defined line on the HR diagram, as several other properties like chemical composition and age influence the location of a star on the diagram.
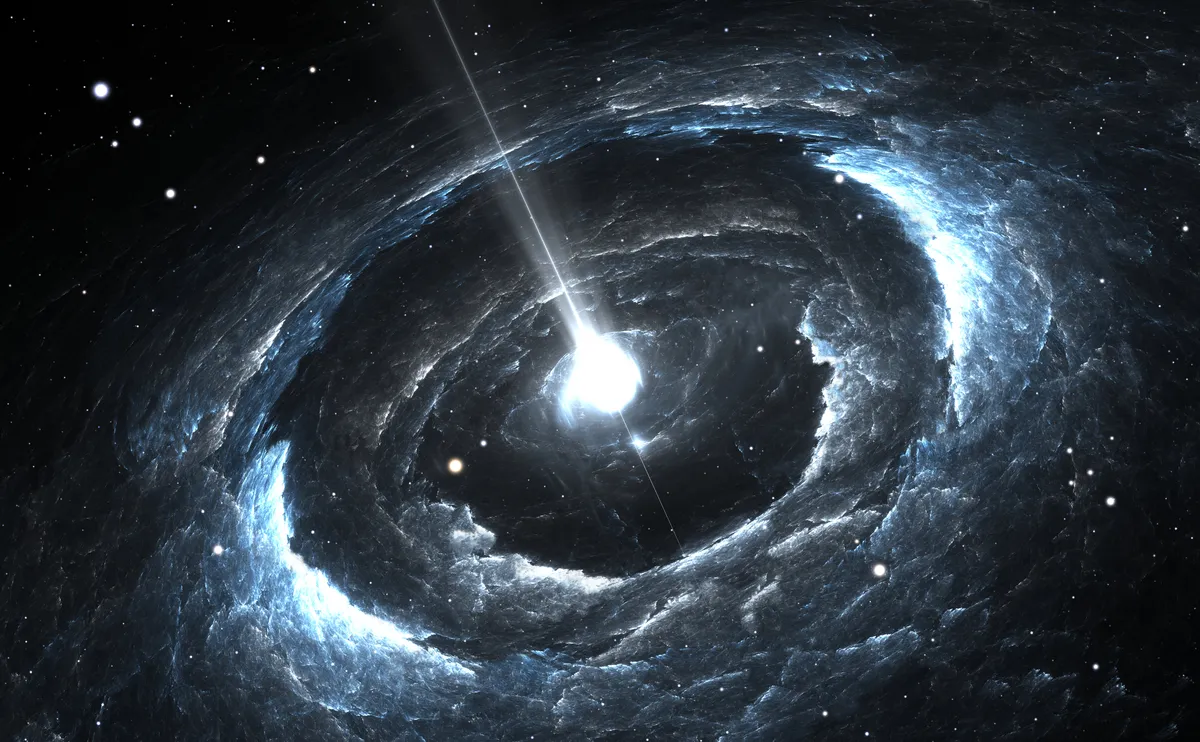
A main sequence star is in a state of hydrostatic equilibrium – the internal pressure created by the nuclear reactions at the star’s core balances the inward pull of gravity, and the star is stable.
But once hydrogen burning in the core ceases, the star loses this stability and evolves away from, or off, the main sequence.
The star swells up and becomes a red giant or supergiant. The surface temperatures of these stars are relatively cool since they are expanding, but they are very luminous, so they are plotted on the HR diagram above and to the right of the main sequence.
Eventually, the star will reach the end point of its life and become a white dwarf, neutron star or black hole – again depending on its mass.
Only white dwarfs are normally plotted on an HR diagram.
2
What is inside the Sun?
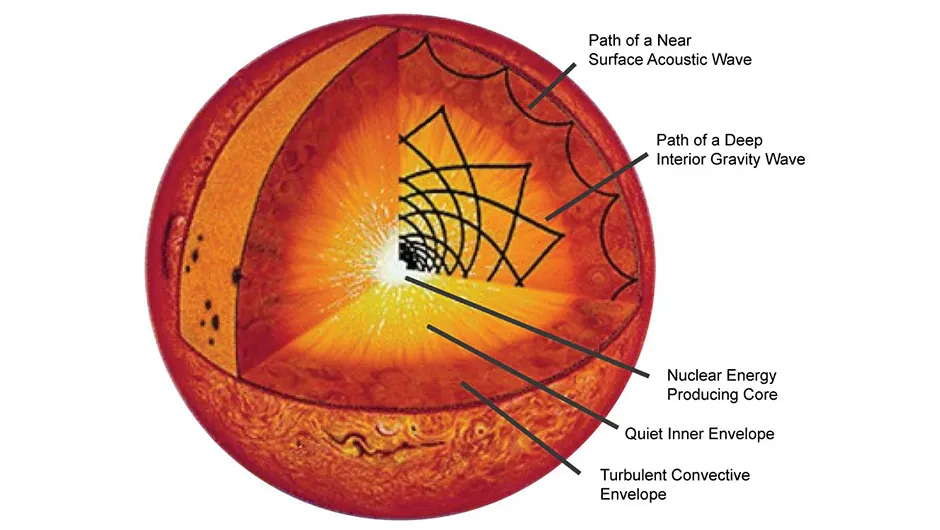
At the centre of the Sun is the core, which produces energy by nuclear fusion, converting hydrogen into helium.
Energy travels into the radiative zone above the core, which typically keeps it trapped for thousands of years.
Above the radiative zone is the convective layer. Making up around 30% of the interior, this transfers energy to the surface (the ‘photosphere’) by the process of convection: gas rises and falls just like blobs of wax in a lava lamp.
The photosphere has been studied using ground-based solar telescopes.
Surrounding the Sun is its atmosphere – the solar corona.
Accurate measurements and studies of the corona have been made by ground-based studies, during solar eclipses and by space-based observatories.
Data from space missions also enables astronomers to measure the vast solar magnetic field, which helps to refine models of our star’s structure.
3
How do we know what's inside the Sun?
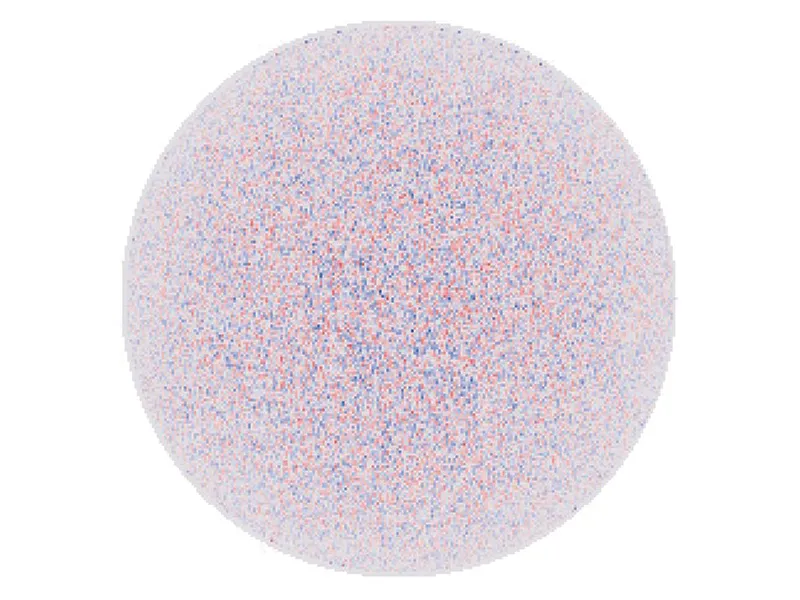
In order to understand the structure of the Sun, astronomers use a mixture of observation, theories and computer modelling to come up with a picture that best describes the characteristics that have been recorded.
Much of our knowledge of the solar interior derives from helioseismology.
Like terrestrial seismologists, helioseismologists use oscillations to probe the interior of a body: seismic waves generated by earthquakes in the case of the Earth; pressure waves within the convective zone in the case of the Sun.
These waves cause vertical motions at the Sun’s surface, the photosphere. Observing the oscillations reveals the properties of the different layers.
The waves within the Sun can be best described as a continuum and many sources produce a constant acoustic ring.
At the solar surface, these waves produce tiny up and down motions in the gases, which can be seen as Doppler shifts in high resolution spectra.
The temperature, composition and other properties of the solar interior strongly influence the behaviour of these waves.
Combining such observations with theories about star structure allows astronomers to produce models of the Sun’s internal composition.
We see three types of waves in the motion of the Sun’s surface: acoustic, gravity and surface gravity waves.
The oscillations have wavelengths ranging from thousands to hundreds of thousands of kilometres.
4
Was the Sun born in a star cluster? Where are the other stars?
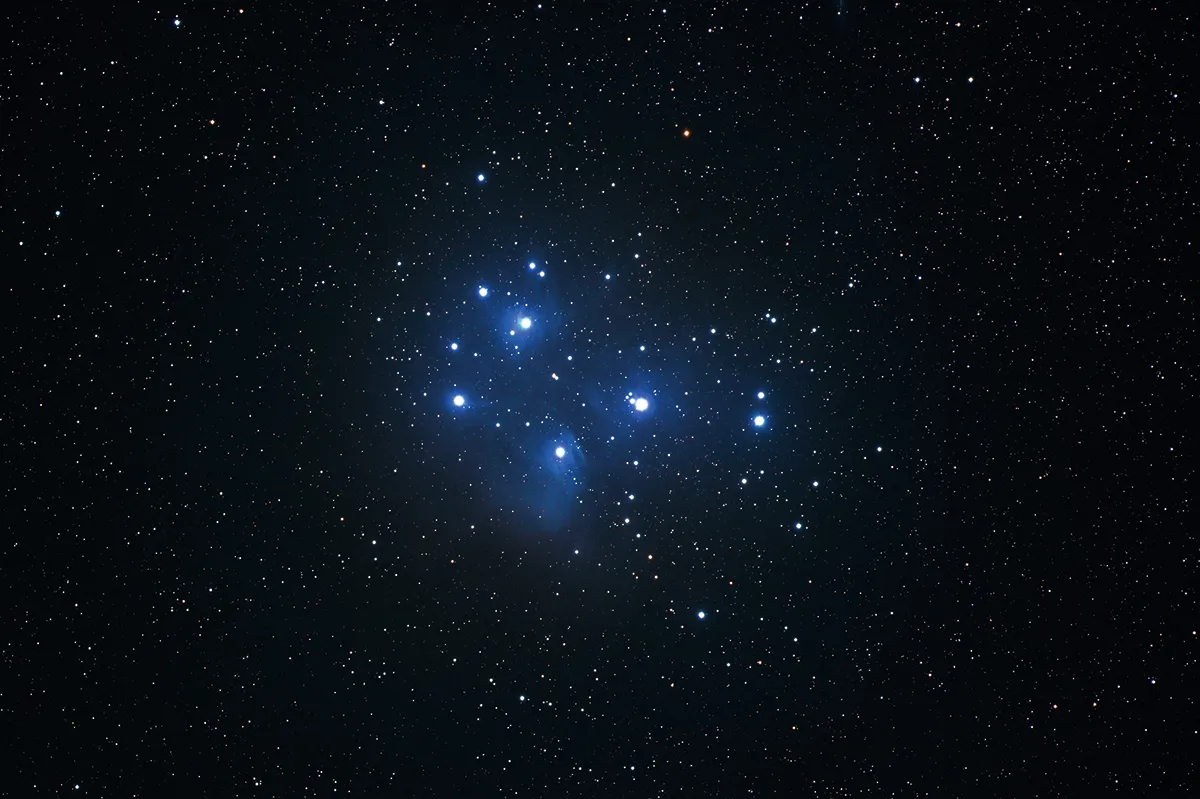
Like most stars that we see in the night sky, the Sun isn’t gravitationally bound to a cluster of stars.
However, astronomers believe that the Sun (like most stars) was born as part of a cluster.
The most massive stars in that cluster would now have exhausted their fuel and died.
Over its 4.6-billion-year lifetime, the Sun has completed around 20 orbits of our Milky Way Galaxy, and the gravitational forces acting on the original cluster members have long since dispersed the Sun’s siblings.
At present, the Sun appears to be located within the Ursa Major Moving Group – a loose group of stars that share the same age, metallicity, radial velocity and proper motion.
However, the Sun isn’t actually a member of this young (approximately 300 million years old) grouping.
5
Where is the Sun located in the Milky Way?
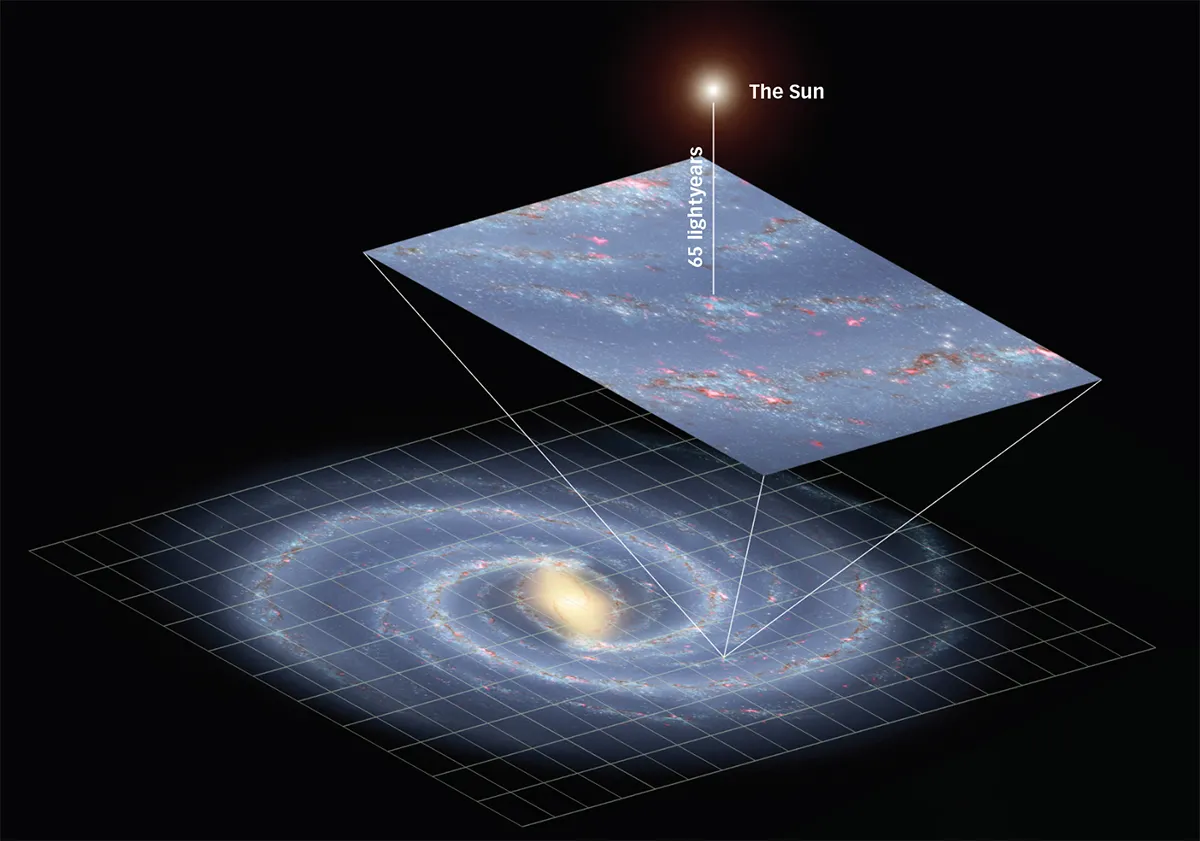
Observations of the distribution of neutral hydrogen gas in the 1960s indicated that our Sun sits somewhere above the mid-plane of our galaxy, the Milky Way.
Since then, a variety of techniques have been used to try and estimate just how far above the galactic plane the Sun actually sits.
Most measurements are based on statistical studies of the distribution of stars, clusters, gas or dust around the Sun, and estimates have ranged from only 6 parsecs (1 parsec is about 3.26 lightyears) to as much as 42 parsecs.
The most recent estimates have suggested a likely distance of around 20 parsecs, or 65 lightyears.
This distance is not constant, however, as the Sun rises and falls as it completes its 230-250 million year orbit around the Galaxy.
It is estimated that the Sun undergoes 2.7 oscillations per ‘galactic year’, with the last passage through the plane being about 3-5 million years ago.
The maximum distance the Sun can get above or below the plane is thought to be 50-100 parsecs (between 163 and 326 lightyears).
6
What heats the Sun's corona?
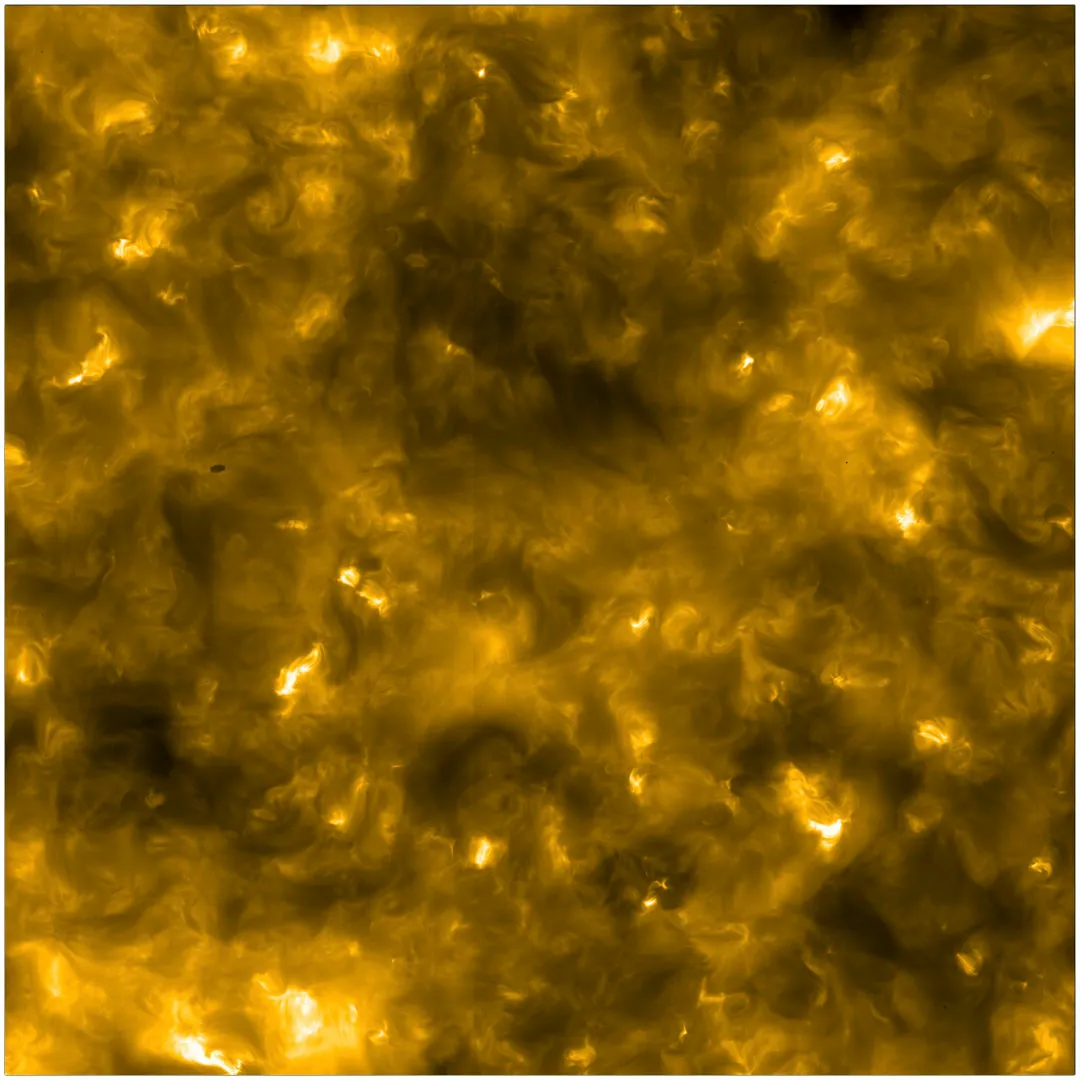
The corona is part of the Sun’s outer atmosphere and is composed of ionised gas.
The Sun’s visible surface (photosphere) has a temperature of about 5,800 Kelvin, but the corona has maximum temperatures between 1 and 3 million Kelvin.
However, the corona is extremely tenuous, with a density only a thousand-billionth that of the photosphere.
It also gives off very little visible light, and can only be seen with a coronagraph or during a total eclipse, when the bright photosphere is hidden from view.
But what makes the corona so hot? There are currently two main theories for the ‘coronal heating problem’.
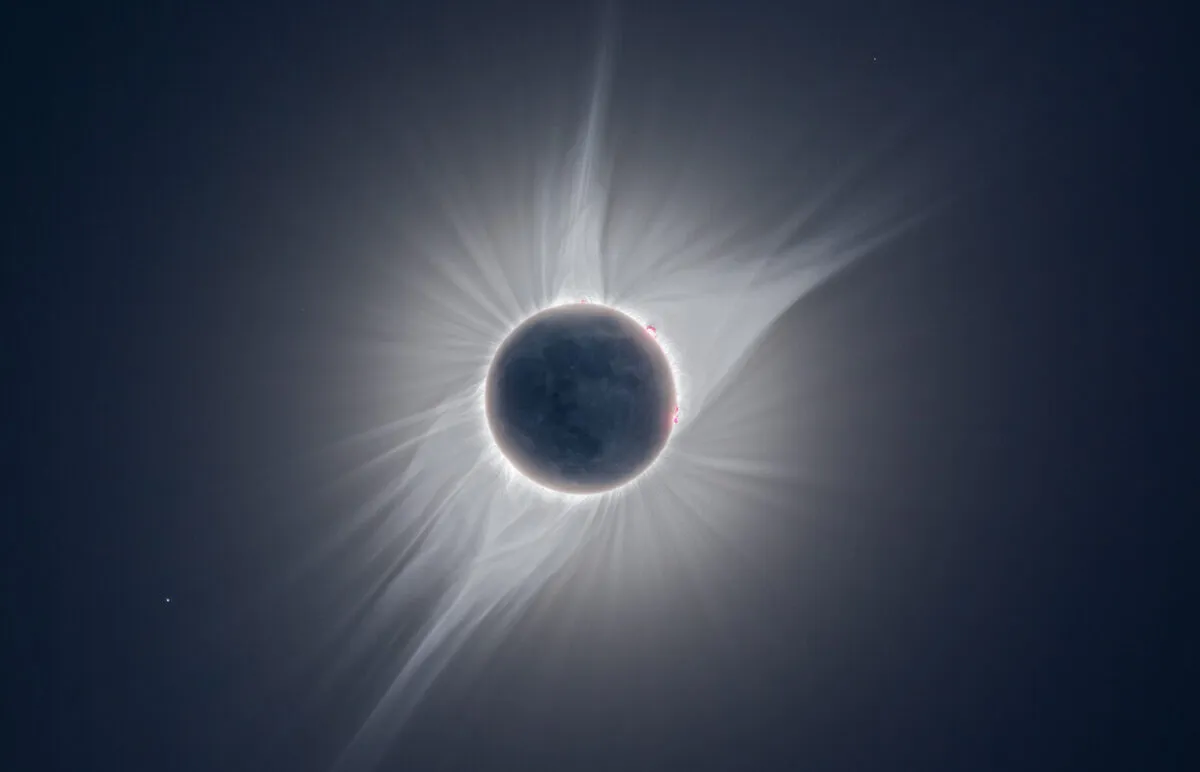
One theory proposes that magneto-acoustic waves (sound waves modified by a magnetic field) and Alfvén waves (ions oscillating in a magnetic field within a plasma) transfer energy from inside the Sun.
Another theory argues that it is rather the Sun’s magnetic field, which induces electrical fields in the corona, that causes the heating.
These fields then collapse suddenly, releasing energy into the corona and heating it.
Theorists and observers are still trying to determine which mechanism is responsible for the coronal heating problem, but it does seem clear that magnetic fields play a key role.
7
What is the Sun butterfly diagram?
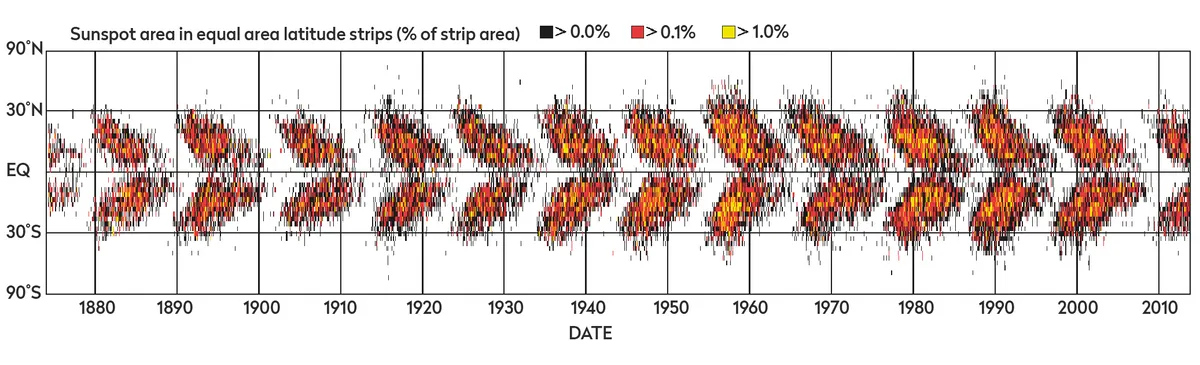
The term ‘butterfly diagram’ refers to the pattern seen on a plot of the latitude of sunspots against time, over many solar cycles.
This time-latitude diagram was first plotted by the husband and wife team of Edward Walter Maunder and Annie Maunder in the early 20th century.
The ‘butterfly’ pattern results from the gradual tendency of sunspots to form at lower latitudes (closer to the equator) as the roughly 11-year sunspot cycle progresses.
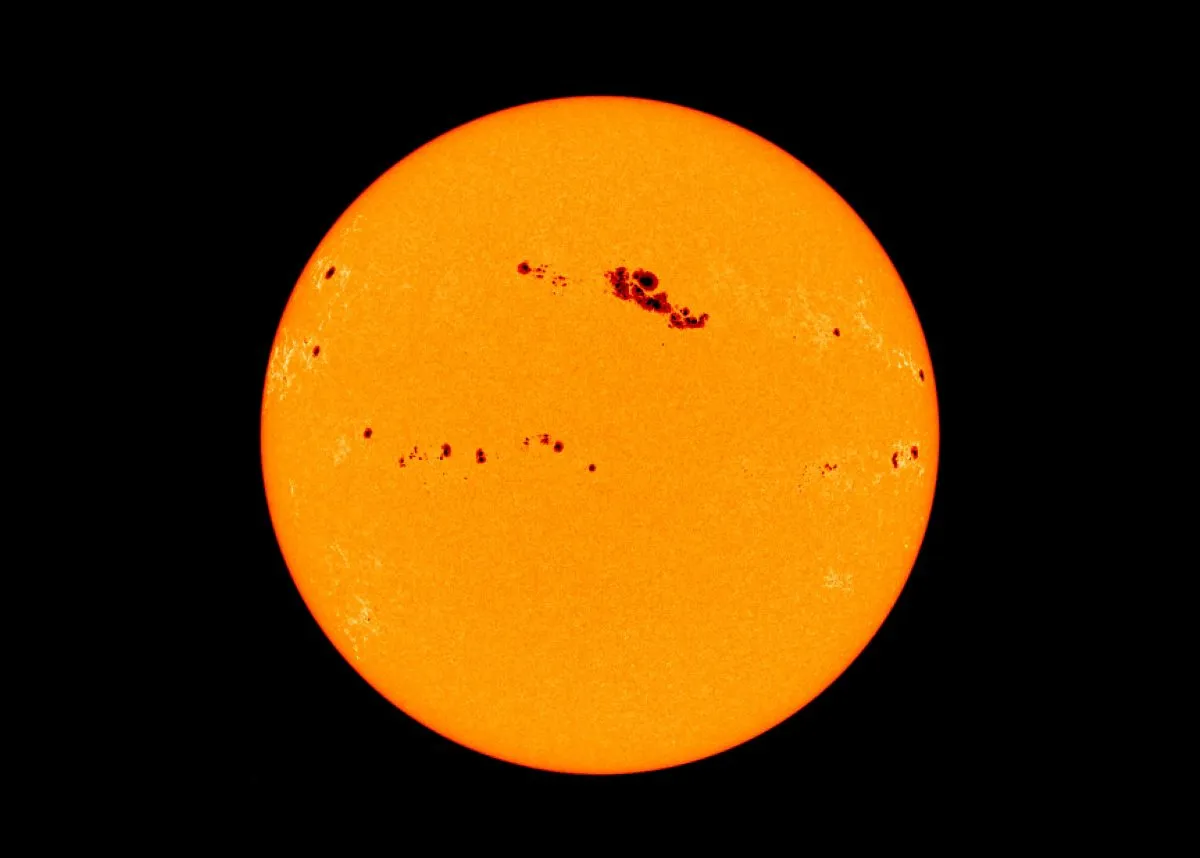
This effect was first noted by the German astronomer Gustav Spörer in the late 19th century.
At the start of a solar cycle (solar minimum), very few sunspots are seen, and these form at high latitudes.
As the number of sunspots increases (heading towards solar maximum), they occur closer and closer to the equator.
After maximum, sunspot numbers decrease again towards the next solar minimum, when the pattern repeats.
The observed pattern reflects the gradual ‘winding up’ of the solar magnetic field.
The field lines become more intensely twisted due to the differential rotation of the Sun’s surface, with the equatorial regions rotating faster than higher latitudes.
The butterfly diagram shows that sunspots are first observed at around latitude 30° north and south.
If you'd like to safely observe these features yourself, read our guide on how to observe sunspots.
8
How close could a crewed spacecraft travel to the Sun?
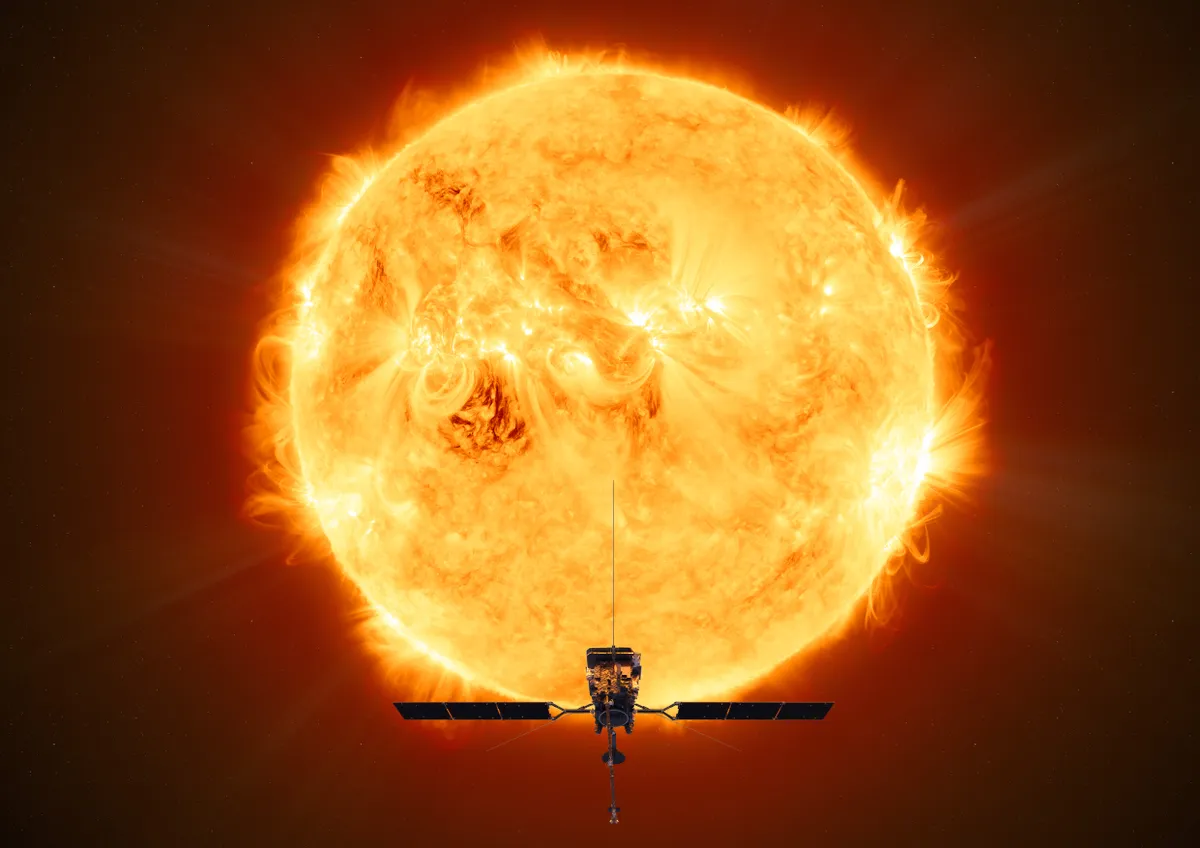
You might think that it would be the problem of cooling a spaceship as it approached the Sun that would be the limiting factor in how close it could travel to the Sun, but thermal protection is a well-developed field.
Current missions like Solar Orbiter use advanced cooling systems combined with highly reflective materials to allow it to operate in the extreme conditions.
Radiation poses a far greater risk for astronauts, even at distances beyond Earth and out to Mars’s orbit.
The Sun’s activity roughly follows an 11-year cycle: there are greater risks of solar storms around the solar maximum.
Astronauts caught outside Earth’s protective magnetosphere during a solar storm can be exposed to lethal doses of radiation in minutes without adequate shielding.

Future missions to Mars could use spacecraft with water-filled walls that would double as a shield from solar radiation.
And launches would be timed to avoid travelling out or back around solar maximum.
Even outside the most dangerous times, living in space is extremely hazardous.
So far, no human has yet travelled further than the far side of the Moon, which is still nearby, even in Solar System terms.
The dangers of long-term exposure to radiation, and even the potential psychological problems of travelling close to the Sun, are still unknown.
So there is no specific distance from the Sun that is considered safe, as even in Earth orbit spacecraft and astronauts can suffer severe damage from our star.
Crewed missions to the innermost planet, Mercury, are therefore highly unlikely, even in the next century.
9
If the Sun is at one point of Earth’s elliptical orbit, what is at the other?
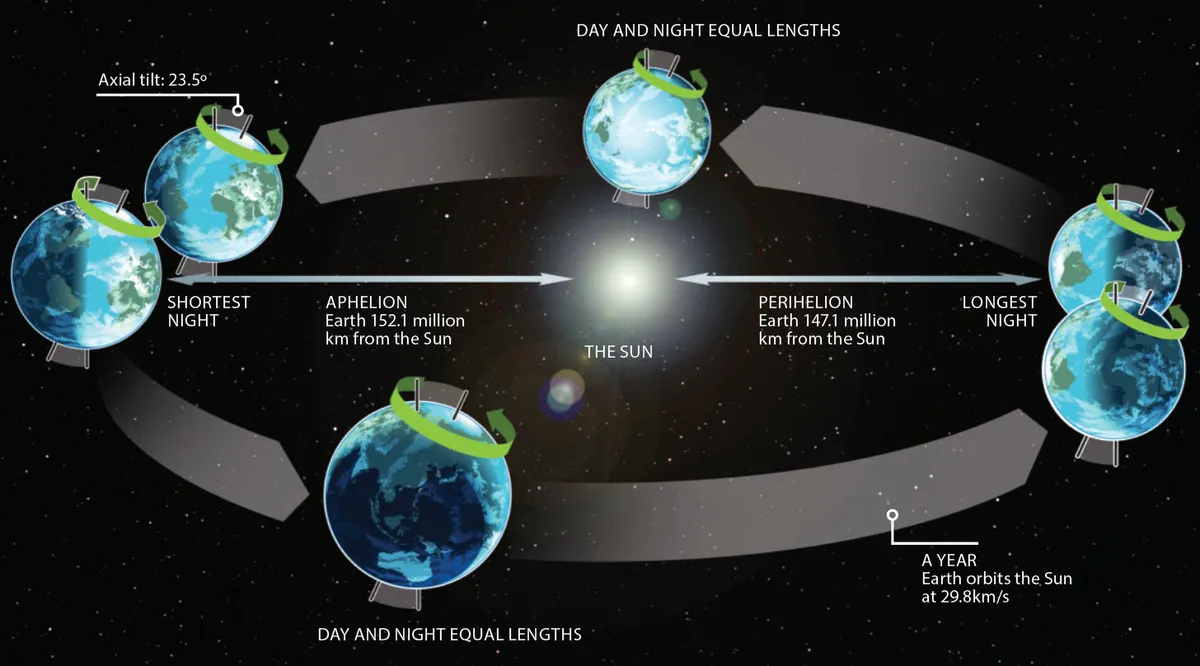
The simple answer is nothing. Misconceptions abound about planetary orbits, and the existence of more than one focus can confuse.
Orbits are elliptical and the foci are nothing more than two points of geometric interest.
They are located either side of the centre of an ellipse, on the major axis, and the sum of the distances from each of these points to the curve of an ellipse is constant.
The Sun and the planets move around each other, with the centre of mass (the barycentre) at one focus of the elliptical orbits.
As the Sun contains 99.9% of the mass of the Solar System, it appears the planets are orbiting the Sun. But the Sun also orbits the barycentre: its orbit is just very small.
For each planet in the Solar system, you could draw a planet-Sun ellipse, each with foci in different locations.
The eccentricity of planetary orbits ranges from almost circular for Venus, to Pluto’s highly elliptical orbit.
In the case of the Earth-Sun system, the barycentre is only 450km (280 miles) from the Sun’s centre.
But if all the planets aligned on the same side, the combined barycentre would be 500,000km (310,000 miles) above the solar surface.
10
What will eventually happen to our Sun?
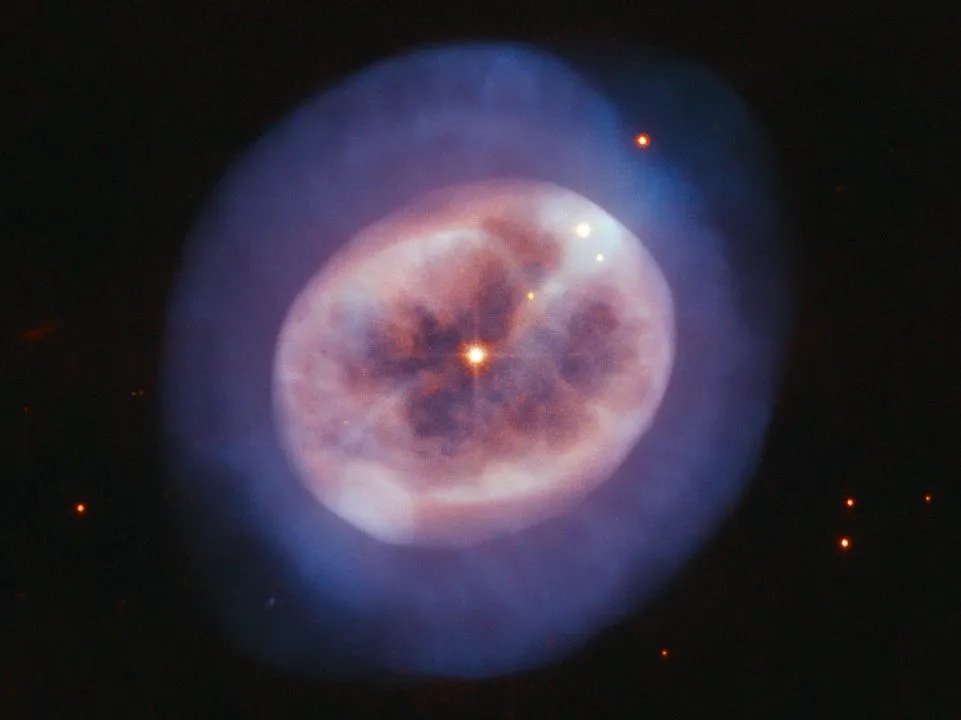
The fate of our Sun, and all stars, is determined by mass.
Despite seeming huge in comparison to Earth, the Sun is fairly average by stellar standards, and will live and die in a fairly unspectacular way – indeed 99% of stars in the Galaxy will end their lives in this manner.
Once the Sun exhausts the hydrogen supplies in its core, it will start burning helium.
This starts a swelling and cooling phase that will leave the Sun as a massive red giant.
The Sun will swell to the point when its outer atmosphere will engulf Mercury and Venus. Earth will have been heated to intolerable levels several billions years before this.
After tens or hundreds of millions of years as a red giant, the Sun will enter an unstable phase.
It will shed its outer atmosphere, which will form a planetary nebula. The exposed core of the Sun will continue to shine at the heart of the expanding nebula, but it will be a white dwarf star, about the size of the Earth.
But there’s no need to panic just yet. It’s estimated the Sun has enough fuel to go on for another five billion years or so.
Even then, if science and technology continue to advance, we may be able to colonise other worlds: home for our descendants may be a planet billions of miles away.